Exoplanet Biosignatures: Understanding Oxygen as a Biosignature in the Context of Its Environment
- PMID: 29746149
- PMCID: PMC6014580
- DOI: 10.1089/ast.2017.1727
Exoplanet Biosignatures: Understanding Oxygen as a Biosignature in the Context of Its Environment
Abstract
We describe how environmental context can help determine whether oxygen (O2) detected in extrasolar planetary observations is more likely to have a biological source. Here we provide an in-depth, interdisciplinary example of O2 biosignature identification and observation, which serves as the prototype for the development of a general framework for biosignature assessment. Photosynthetically generated O2 is a potentially strong biosignature, and at high abundance, it was originally thought to be an unambiguous indicator for life. However, as a biosignature, O2 faces two major challenges: (1) it was only present at high abundance for a relatively short period of Earth's history and (2) we now know of several potential planetary mechanisms that can generate abundant O2 without life being present. Consequently, our ability to interpret both the presence and absence of O2 in an exoplanetary spectrum relies on understanding the environmental context. Here we examine the coevolution of life with the early Earth's environment to identify how the interplay of sources and sinks may have suppressed O2 release into the atmosphere for several billion years, producing a false negative for biologically generated O2. These studies suggest that planetary characteristics that may enhance false negatives should be considered when selecting targets for biosignature searches. We review the most recent knowledge of false positives for O2, planetary processes that may generate abundant atmospheric O2 without a biosphere. We provide examples of how future photometric, spectroscopic, and time-dependent observations of O2 and other aspects of the planetary environment can be used to rule out false positives and thereby increase our confidence that any observed O2 is indeed a biosignature. These insights will guide and inform the development of future exoplanet characterization missions. Key Words: Biosignatures-Oxygenic photosynthesis-Exoplanets-Planetary atmospheres. Astrobiology 18, 630-662.
Conflict of interest statement
No competing financial interests exist.
Figures
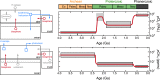
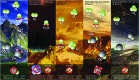
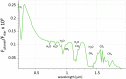
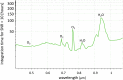
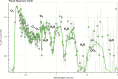
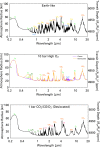
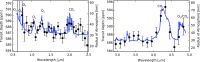
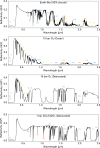
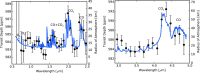
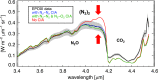

Similar articles
-
Reflections on O2 as a Biosignature in Exoplanetary Atmospheres.Astrobiology. 2017 Oct;17(10):1022-1052. doi: 10.1089/ast.2016.1578. Epub 2017 Apr 26. Astrobiology. 2017. PMID: 28443722 Free PMC article.
-
False Negatives for Remote Life Detection on Ocean-Bearing Planets: Lessons from the Early Earth.Astrobiology. 2017 Apr;17(4):287-297. doi: 10.1089/ast.2016.1598. Astrobiology. 2017. PMID: 28418704 Free PMC article.
-
Exoplanet Biosignatures: At the Dawn of a New Era of Planetary Observations.Astrobiology. 2018 Jun;18(6):619-629. doi: 10.1089/ast.2018.1862. Epub 2018 May 9. Astrobiology. 2018. PMID: 29741918 Free PMC article.
-
Exoplanet Biosignatures: A Framework for Their Assessment.Astrobiology. 2018 Jun;18(6):709-738. doi: 10.1089/ast.2017.1737. Epub 2018 Apr 20. Astrobiology. 2018. PMID: 29676932 Free PMC article. Review.
-
Exoplanet Biosignatures: A Review of Remotely Detectable Signs of Life.Astrobiology. 2018 Jun;18(6):663-708. doi: 10.1089/ast.2017.1729. Epub 2018 May 4. Astrobiology. 2018. PMID: 29727196 Free PMC article. Review.
Cited by
-
A 600 m2 array of 6.5 m telescopes at the lunar pole.Philos Trans A Math Phys Eng Sci. 2024 May 9;382(2271):20230076. doi: 10.1098/rsta.2023.0076. Epub 2024 Mar 25. Philos Trans A Math Phys Eng Sci. 2024. PMID: 38522467 Free PMC article.
-
Instrumentation prospects for rocky exoplanet atmospheres studies with high resolution spectroscopy.Sci Rep. 2024 Nov 9;14(1):27356. doi: 10.1038/s41598-024-78071-5. Sci Rep. 2024. PMID: 39521809 Free PMC article.
-
Exoplanet Biosignatures: Observational Prospects.Astrobiology. 2018 Jun;18(6):739-778. doi: 10.1089/ast.2017.1733. Astrobiology. 2018. PMID: 29938537 Free PMC article. Review.
-
Can Isotopologues Be Used as Biosignature Gases in Exoplanet Atmospheres?Life (Basel). 2023 Dec 11;13(12):2325. doi: 10.3390/life13122325. Life (Basel). 2023. PMID: 38137926 Free PMC article. Review.
-
Evaluating Biogenicity on the Geological Record With Synchrotron-Based Techniques.Front Microbiol. 2019 Oct 11;10:2358. doi: 10.3389/fmicb.2019.02358. eCollection 2019. Front Microbiol. 2019. PMID: 31681221 Free PMC article. Review.
References
-
- Airapetian V.S., Glocer A., Gronoff G., Hébrard E., and Danchi W. (2016) Prebiotic chemistry and atmospheric warming of early Earth by an active young Sun. Nat Geosci 9:52
-
- Airapetian V.S., Glocer A., Khazanov G.V., Loyd R.O.P., France K., Sojka J., Danchi W.C., and Liemohn M.W. (2017) How hospitable are space weather affected habitable zones? The role of ion escape. Astrophys J Lett 836:L3
-
- Allen J.P. and Williams J.C. (1998) Photosynthetic reaction centers. FEBS Lett 438:5–9 - PubMed
-
- Anbar A.D., Duan Y., Lyons T.W., Arnold G.L., Kendall B., Creaser R.A., Kaufman A.J., Gordon G.W., Scott C., Garvin J., and Buick R. (2007) A whiff of oxygen before the great oxidation event? Science (New York, N.Y.) 317:1903–1906 - PubMed
-
- Anglada-Escudé G., Amado P.J., Barnes J., Berdiñas Z.M., Butler R.P., Coleman G.A.L., de la Cueva I., Dreizler S., Endl M., Giesers B., Jeffers S.V., Jenkins J.S., Jones H.R.A., Kiraga M., Kürster M., López-González M.J., Marvin C.J., Morales N., Morin J., Nelson R.P., Ortiz J.L., Ofir A., Paardekooper S.-J., Reiners A., Rodríguez E., Rodríguez-López C., Sarmiento L.F., Strachan J.P., Tsapras Y., Tuomi M., and Zechmeister M. (2016) A terrestrial planet candidate in a temperate orbit around Proxima Centauri. Nature 536:437–440 - PubMed
Publication types
MeSH terms
Substances
LinkOut - more resources
Full Text Sources
Other Literature Sources