Sterol Synthesis in Diverse Bacteria
- PMID: 27446030
- PMCID: PMC4919349
- DOI: 10.3389/fmicb.2016.00990
Sterol Synthesis in Diverse Bacteria
Abstract
Sterols are essential components of eukaryotic cells whose biosynthesis and function has been studied extensively. Sterols are also recognized as the diagenetic precursors of steranes preserved in sedimentary rocks where they can function as geological proxies for eukaryotic organisms and/or aerobic metabolisms and environments. However, production of these lipids is not restricted to the eukaryotic domain as a few bacterial species also synthesize sterols. Phylogenomic studies have identified genes encoding homologs of sterol biosynthesis proteins in the genomes of several additional species, indicating that sterol production may be more widespread in the bacterial domain than previously thought. Although the occurrence of sterol synthesis genes in a genome indicates the potential for sterol production, it provides neither conclusive evidence of sterol synthesis nor information about the composition and abundance of basic and modified sterols that are actually being produced. Here, we coupled bioinformatics with lipid analyses to investigate the scope of bacterial sterol production. We identified oxidosqualene cyclase (Osc), which catalyzes the initial cyclization of oxidosqualene to the basic sterol structure, in 34 bacterial genomes from five phyla (Bacteroidetes, Cyanobacteria, Planctomycetes, Proteobacteria, and Verrucomicrobia) and in 176 metagenomes. Our data indicate that bacterial sterol synthesis likely occurs in diverse organisms and environments and also provides evidence that there are as yet uncultured groups of bacterial sterol producers. Phylogenetic analysis of bacterial and eukaryotic Osc sequences confirmed a complex evolutionary history of sterol synthesis in this domain. Finally, we characterized the lipids produced by Osc-containing bacteria and found that we could generally predict the ability to synthesize sterols. However, predicting the final modified sterol based on our current knowledge of sterol synthesis was difficult. Some bacteria produced demethylated and saturated sterol products even though they lacked homologs of the eukaryotic proteins required for these modifications emphasizing that several aspects of bacterial sterol synthesis are still completely unknown.
Keywords: biomarkers; lipid biosynthesis; methanotrophs; myxobacteria; oxidosqualene cyclase; planctomycetes; squalene epoxidase; sterols.
Figures
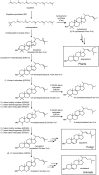
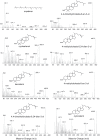
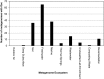
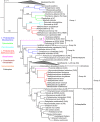
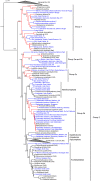
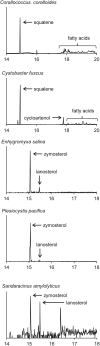
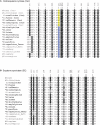
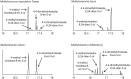
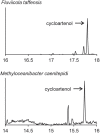
Similar articles
-
C-4 sterol demethylation enzymes distinguish bacterial and eukaryotic sterol synthesis.Proc Natl Acad Sci U S A. 2018 Jun 5;115(23):5884-5889. doi: 10.1073/pnas.1802930115. Epub 2018 May 21. Proc Natl Acad Sci U S A. 2018. PMID: 29784781 Free PMC article.
-
Phylogenetic and biochemical evidence for sterol synthesis in the bacterium Gemmata obscuriglobus.Proc Natl Acad Sci U S A. 2003 Dec 23;100(26):15352-7. doi: 10.1073/pnas.2536559100. Epub 2003 Dec 5. Proc Natl Acad Sci U S A. 2003. PMID: 14660793 Free PMC article.
-
Paleoproterozoic sterol biosynthesis and the rise of oxygen.Nature. 2017 Mar 16;543(7645):420-423. doi: 10.1038/nature21412. Epub 2017 Mar 6. Nature. 2017. PMID: 28264195
-
Incomplete sterols and hopanoids pathways in ciliates: gene loss and acquisition during evolution as a source of biosynthetic genes.Mol Phylogenet Evol. 2014 May;74:122-34. doi: 10.1016/j.ympev.2014.01.026. Epub 2014 Feb 10. Mol Phylogenet Evol. 2014. PMID: 24525200 Review.
-
Noncholesterol sterols.Acta Univ Carol Med Monogr. 2008;154:5-101. Acta Univ Carol Med Monogr. 2008. PMID: 19283968 Review.
Cited by
-
Novel sterol binding domains in bacteria.Elife. 2024 Feb 8;12:RP90696. doi: 10.7554/eLife.90696. Elife. 2024. PMID: 38329015 Free PMC article.
-
Impact of steroid biosynthesis on the aerobic adaptation of eukaryotes.Geobiology. 2024 Jul-Aug;22(4):e12612. doi: 10.1111/gbi.12612. Geobiology. 2024. PMID: 38967402 Review.
-
Free and kerogen-bound biomarkers from late Tonian sedimentary rocks record abundant eukaryotes in mid-Neoproterozoic marine communities.Geobiology. 2020 May;18(3):326-347. doi: 10.1111/gbi.12378. Epub 2019 Dec 21. Geobiology. 2020. PMID: 31865640 Free PMC article.
-
Metagenomics and Faecal Metabolomics Integrative Analysis towards the Impaired Glucose Regulation and Type 2 Diabetes in Uyghur-Related Omics.J Diabetes Res. 2019 Nov 18;2019:2893041. doi: 10.1155/2019/2893041. eCollection 2019. J Diabetes Res. 2019. PMID: 31828159 Free PMC article.
-
Efficient conversion of phytosterols into 4-androstene-3,17-dione and its C1,2-dehydrogenized and 9α-hydroxylated derivatives by engineered Mycobacteria.Microb Cell Fact. 2021 Aug 16;20(1):158. doi: 10.1186/s12934-021-01653-9. Microb Cell Fact. 2021. PMID: 34399754 Free PMC article.
References
LinkOut - more resources
Full Text Sources
Other Literature Sources
Molecular Biology Databases