The Habitability of Proxima Centauri b: Environmental States and Observational Discriminants
- PMID: 29431479
- PMCID: PMC5820795
- DOI: 10.1089/ast.2016.1589
The Habitability of Proxima Centauri b: Environmental States and Observational Discriminants
Abstract
Proxima Centauri b provides an unprecedented opportunity to understand the evolution and nature of terrestrial planets orbiting M dwarfs. Although Proxima Cen b orbits within its star's habitable zone, multiple plausible evolutionary paths could have generated different environments that may or may not be habitable. Here, we use 1-D coupled climate-photochemical models to generate self-consistent atmospheres for several evolutionary scenarios, including high-O2, high-CO2, and more Earth-like atmospheres, with both oxic and anoxic compositions. We show that these modeled environments can be habitable or uninhabitable at Proxima Cen b's position in the habitable zone. We use radiative transfer models to generate synthetic spectra and thermal phase curves for these simulated environments, and use instrument models to explore our ability to discriminate between possible planetary states. These results are applicable not only to Proxima Cen b but to other terrestrial planets orbiting M dwarfs. Thermal phase curves may provide the first constraint on the existence of an atmosphere. We find that James Webb Space Telescope (JWST) observations longward of 10 μm could characterize atmospheric heat transport and molecular composition. Detection of ocean glint is unlikely with JWST but may be within the reach of larger-aperture telescopes. Direct imaging spectra may detect O4 absorption, which is diagnostic of massive water loss and O2 retention, rather than a photosynthetic biosphere. Similarly, strong CO2 and CO bands at wavelengths shortward of 2.5 μm would indicate a CO2-dominated atmosphere. If the planet is habitable and volatile-rich, direct imaging will be the best means of detecting habitability. Earth-like planets with microbial biospheres may be identified by the presence of CH4-which has a longer atmospheric lifetime under Proxima Centauri's incident UV-and either photosynthetically produced O2 or a hydrocarbon haze layer. Key Words: Planetary habitability and biosignatures-Planetary atmospheres-Exoplanets-Spectroscopic biosignatures-Planetary science-Proxima Centauri b. Astrobiology 18, 133-189.
Conflict of interest statement
No competing financial interests exist.
Figures
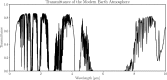
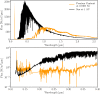
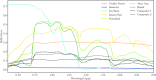
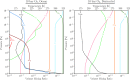
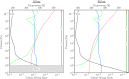
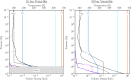
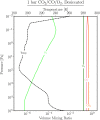
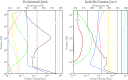
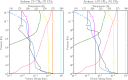
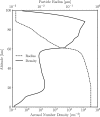
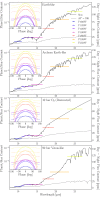
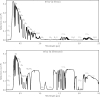
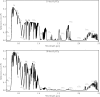
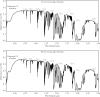
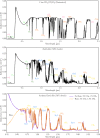
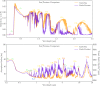
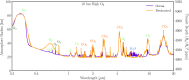
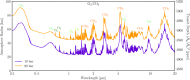
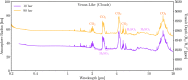
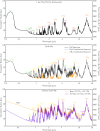
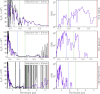
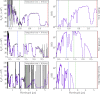
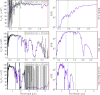
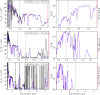
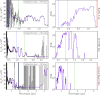
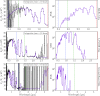
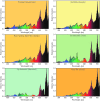
Similar articles
-
Reflections on O2 as a Biosignature in Exoplanetary Atmospheres.Astrobiology. 2017 Oct;17(10):1022-1052. doi: 10.1089/ast.2016.1578. Epub 2017 Apr 26. Astrobiology. 2017. PMID: 28443722 Free PMC article.
-
Evolution of Earth-like Extrasolar Planetary Atmospheres: Assessing the Atmospheres and Biospheres of Early Earth Analog Planets with a Coupled Atmosphere Biogeochemical Model.Astrobiology. 2017 Jan;17(1):27-54. doi: 10.1089/ast.2015.1384. Astrobiology. 2017. PMID: 28103105
-
Habitable Climate Scenarios for Proxima Centauri b with a Dynamic Ocean.Astrobiology. 2019 Jan;19(1):99-125. doi: 10.1089/ast.2017.1760. Epub 2018 Sep 5. Astrobiology. 2019. PMID: 30183335
-
M stars as targets for terrestrial exoplanet searches and biosignature detection.Astrobiology. 2007 Feb;7(1):85-166. doi: 10.1089/ast.2006.0125. Astrobiology. 2007. PMID: 17407405 Review.
-
Exoplanet Biosignatures: Observational Prospects.Astrobiology. 2018 Jun;18(6):739-778. doi: 10.1089/ast.2017.1733. Astrobiology. 2018. PMID: 29938537 Free PMC article. Review.
Cited by
-
Synergies Between Venus & Exoplanetary Observations: Venus and Its Extrasolar Siblings.Space Sci Rev. 2023;219(1):13. doi: 10.1007/s11214-023-00953-3. Epub 2023 Feb 9. Space Sci Rev. 2023. PMID: 36785654 Free PMC article. Review.
-
Exoplanet Biosignatures: Understanding Oxygen as a Biosignature in the Context of Its Environment.Astrobiology. 2018 Jun;18(6):630-662. doi: 10.1089/ast.2017.1727. Epub 2018 May 10. Astrobiology. 2018. PMID: 29746149 Free PMC article. Review.
-
Disequilibrium biosignatures over Earth history and implications for detecting exoplanet life.Sci Adv. 2018 Jan 24;4(1):eaao5747. doi: 10.1126/sciadv.aao5747. eCollection 2018 Jan. Sci Adv. 2018. PMID: 29387792 Free PMC article.
-
Modeling Repeated M Dwarf Flaring at an Earth-like Planet in the Habitable Zone: Atmospheric Effects for an Unmagnetized Planet.Astrobiology. 2019 Jan;19(1):64-86. doi: 10.1089/ast.2017.1794. Epub 2018 Aug 2. Astrobiology. 2019. PMID: 30070900 Free PMC article.
-
Factoring Origin of Life Hypotheses into the Search for Life in the Solar System and Beyond.Life (Basel). 2020 Apr 27;10(5):52. doi: 10.3390/life10050052. Life (Basel). 2020. PMID: 32349245 Free PMC article. Review.
References
-
- Airapetian V.S., Glocer A., Khazanov G.V., Loyd R.O.P., France K., Sojka J., Danchi W.C., and Liemohn M.W. (2017) How hospitable are space weather affected habitable zones? The role of ion escape. Astrophys J 836:L3
-
- Albarède F. (2009) Volatile accretion history of the terrestrial planets and dynamic implications. Nature 461:1227–1233 - PubMed
-
- Anglada-Escudé G., Amado P.J., Barnes J., Berdiñas Z.M., Butler R.P., Coleman G.A., de la Cueva I., Dreizler S., Endl M., Giesers B. J.effers S.V., Jenkins J.S., Jones H.R., Kiraga M., Kürster M., López-González M.J., Marvin C.J., Morales N., Morin J., Nelson R.P., Oritz J.L., Ofir A., Paardekooper S.-J., Reiners A., Rodríguez E., Rodríguez-López C., Sarmiento L.F., Strachan J.P., Tsapras Y., Tuomi M., and Zechmeister M. (2016) A terrestrial planet candidate in a temperate orbit around Proxima Centauri. Nature 536:437–440 - PubMed
-
- Arney G., Meadows V., Crisp D., Schmidt S.J., Bailey J., and Robinson T. (2014) Spatially resolved measurements of H2O, HCl, CO, OCS, SO2, cloud opacity, and acid concentration in the Venus near-infrared spectral windows. J Geophys Res: Planets 119:1860–1891
Publication types
MeSH terms
Substances
LinkOut - more resources
Full Text Sources
Other Literature Sources