Hierarchical organization of bone in three dimensions: A twist of twists
- PMID: 35072054
- PMCID: PMC8762463
- DOI: 10.1016/j.yjsbx.2021.100057
Hierarchical organization of bone in three dimensions: A twist of twists
Abstract
Structural hierarchy of bone - observed across multiple scales and in three dimensions (3D) - is essential to its mechanical performance. While the mineralized extracellular matrix of bone consists predominantly of carbonate-substituted hydroxyapatite, type I collagen fibrils, water, and noncollagenous organic constituents (mainly proteins and small proteoglycans), it is largely the 3D arrangement of these inorganic and organic constituents at each length scale that endow bone with its exceptional mechanical properties. Focusing on recent volumetric imaging studies of bone at each of these scales - from the level of individual mineralized collagen fibrils to that of whole bones - this graphical review builds upon and re-emphasizes the original work of James Bell Pettigrew and D'Arcy Thompson who first described the ubiquity of spiral structure in Nature. Here we illustrate and discuss the omnipresence of twisted, curved, sinusoidal, coiled, spiraling, and braided motifs in bone in at least nine of its twelve hierarchical levels - a visualization undertaking that has not been possible until recently with advances in 3D imaging technologies (previous 2D imaging does not provide this information). From this perspective, we hypothesize that the twisting motif occurring across each hierarchical level of bone is directly linked to enhancement of function, rather than being simply an energetically favorable way to assemble mineralized matrix components. We propose that attentive consideration of twists in bone and the skeleton at different scales will likely develop, and will enhance our understanding of structure-function relationships in bone.
Keywords: Biomineralization; Bone; Electron Microscopy; Helicoidal Structures; Hierarchical Organization; Review; Skeleton; Volume Microscopy and Tomography.
© 2021 The Authors.
Conflict of interest statement
The authors declare that they have no competing financial interests or personal relationships that could appear to have influenced the work reported in this paper.
Figures
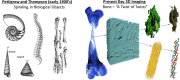
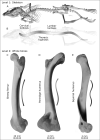
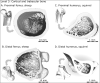
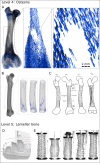
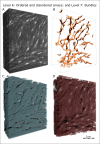
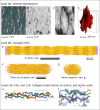
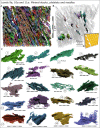
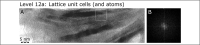
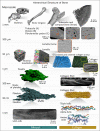
Comment in
-
Announcement: Journal of Structural Biology: X - Paper of the Year.J Struct Biol X. 2024 Oct 30;10:100116. doi: 10.1016/j.yjsbx.2024.100116. eCollection 2024 Dec. J Struct Biol X. 2024. PMID: 39764352 Free PMC article. No abstract available.
Similar articles
-
Dynamic Field Theory of Executive Function: Identifying Early Neurocognitive Markers.Monogr Soc Res Child Dev. 2024 Dec;89(3):7-109. doi: 10.1111/mono.12478. Monogr Soc Res Child Dev. 2024. PMID: 39628288 Free PMC article.
-
RETRACTED: Hydroxychloroquine and azithromycin as a treatment of COVID-19: results of an open-label non-randomized clinical trial.Int J Antimicrob Agents. 2020 Jul;56(1):105949. doi: 10.1016/j.ijantimicag.2020.105949. Epub 2020 Mar 20. Int J Antimicrob Agents. 2020. Retraction in: Int J Antimicrob Agents. 2025 Jan;65(1):107416. doi: 10.1016/j.ijantimicag.2024.107416. PMID: 32205204 Free PMC article. Retracted. Clinical Trial.
-
Far Posterior Approach for Rib Fracture Fixation: Surgical Technique and Tips.JBJS Essent Surg Tech. 2024 Dec 6;14(4):e23.00094. doi: 10.2106/JBJS.ST.23.00094. eCollection 2024 Oct-Dec. JBJS Essent Surg Tech. 2024. PMID: 39650795 Free PMC article.
-
Impact of residual disease as a prognostic factor for survival in women with advanced epithelial ovarian cancer after primary surgery.Cochrane Database Syst Rev. 2022 Sep 26;9(9):CD015048. doi: 10.1002/14651858.CD015048.pub2. Cochrane Database Syst Rev. 2022. PMID: 36161421 Free PMC article. Review.
-
Trends in Surgical and Nonsurgical Aesthetic Procedures: A 14-Year Analysis of the International Society of Aesthetic Plastic Surgery-ISAPS.Aesthetic Plast Surg. 2024 Oct;48(20):4217-4227. doi: 10.1007/s00266-024-04260-2. Epub 2024 Aug 5. Aesthetic Plast Surg. 2024. PMID: 39103642 Review.
Cited by
-
Characterization of human trabecular bone across multiple length scales using a correlative approach combining X-ray tomography with LaserFIB and plasma FIB-SEM.Sci Rep. 2024 Sep 16;14(1):21604. doi: 10.1038/s41598-024-72739-8. Sci Rep. 2024. PMID: 39285214 Free PMC article.
-
Assessment of submicron bone tissue composition in plastic-embedded samples using optical photothermal infrared (O-PTIR) spectral imaging and machine learning.J Struct Biol X. 2024 Oct 9;10:100111. doi: 10.1016/j.yjsbx.2024.100111. eCollection 2024 Dec. J Struct Biol X. 2024. PMID: 39507318 Free PMC article.
-
Twisted-plywood-like tissue formation in vitro. Does curvature do the twist?PNAS Nexus. 2024 Mar 21;3(4):pgae121. doi: 10.1093/pnasnexus/pgae121. eCollection 2024 Apr. PNAS Nexus. 2024. PMID: 38590971 Free PMC article.
-
Organoids in endocrine and metabolic research: current and emerging applications.Nat Rev Endocrinol. 2024 Apr;20(4):195-201. doi: 10.1038/s41574-023-00933-1. Epub 2024 Jan 5. Nat Rev Endocrinol. 2024. PMID: 38182746 No abstract available.
-
Bone Tissue Engineering through 3D Bioprinting of Bioceramic Scaffolds: A Review and Update.Life (Basel). 2022 Jun 16;12(6):903. doi: 10.3390/life12060903. Life (Basel). 2022. PMID: 35743934 Free PMC article. Review.
References
-
- Ayoubi M., Tol A.F., Weinkamer R., Roschger P., Brugger P.C., Berzlanovich A., Bertinetti L., Roschger A., Fratzl P. 3D interrelationship between osteocyte network and forming mineral during human bone remodeling. Adv. Healthcare Mater. 2021;10(12):2100113. doi: 10.1002/adhm.v10.1210.1002/adhm.202100113. - DOI - PMC - PubMed
-
- Boyde A., Hobdell M.H. Scanning electron microscopy of lamellar bone. Z. Zellforsch. 1968;93(2):213–231. - PubMed
-
- Boyde A., Jones S. Aspects of anatomy and development of bone. The nm, um and mm hierarchy. Adv. Organ Biol. 1998;5A:3–44.
Publication types
LinkOut - more resources
Full Text Sources