Construction of an octosyl acid backbone catalyzed by a radical S-adenosylmethionine enzyme and a phosphatase in the biosynthesis of high-carbon sugar nucleoside antibiotics
- PMID: 28451191
- PMCID: PMC5365060
- DOI: 10.1039/c6sc01826b
Construction of an octosyl acid backbone catalyzed by a radical S-adenosylmethionine enzyme and a phosphatase in the biosynthesis of high-carbon sugar nucleoside antibiotics
Abstract
Unique bicyclic octosyl uronic acid nucleosides include ezomycin, malayamycin, and octosyl acid (OA). They are structurally characterized by OA, an unusual 8-carbon furanosyl nucleoside core proposed to be the precursor to polyoxin and nikkomycin. Despite the well-known bioactivity of these nucleoside antibiotics, the biosynthesis of OA has not been elucidated yet. Here we report the two pivotal enzymatic steps in the polyoxin biosynthetic pathway leading to the identification of OA as a key intermediate. Our data suggest that this intermediate is formed via a free radical reaction catalyzed by the radical S-adenosylmethionine (SAM) enzyme, PolH, and using 3'-enolpyruvyl uridine 5'-monophosphate (3'-EUMP) as a substrate. Subsequent dephosphorylation catalyzed by phosphatase PolJ converts the resulting octosyl acid 5'-phosphate (OAP) to OA. These results provide, for the first time, significant in vitro evidence for the biosynthetic origins of the C8 backbone of OA.
Figures
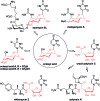
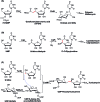
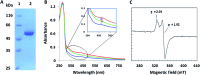
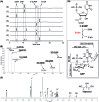
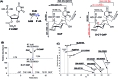
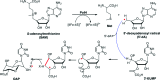
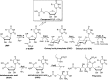
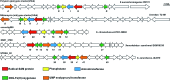
Similar articles
-
RETRACTED: Hydroxychloroquine and azithromycin as a treatment of COVID-19: results of an open-label non-randomized clinical trial.Int J Antimicrob Agents. 2020 Jul;56(1):105949. doi: 10.1016/j.ijantimicag.2020.105949. Epub 2020 Mar 20. Int J Antimicrob Agents. 2020. Retraction in: Int J Antimicrob Agents. 2025 Jan;65(1):107416. doi: 10.1016/j.ijantimicag.2024.107416. PMID: 32205204 Free PMC article. Retracted. Clinical Trial.
-
Interventions to reduce harm from continued tobacco use.Cochrane Database Syst Rev. 2016 Oct 13;10(10):CD005231. doi: 10.1002/14651858.CD005231.pub3. Cochrane Database Syst Rev. 2016. PMID: 27734465 Free PMC article. Review.
-
Using Experience Sampling Methodology to Capture Disclosure Opportunities for Autistic Adults.Autism Adulthood. 2023 Dec 1;5(4):389-400. doi: 10.1089/aut.2022.0090. Epub 2023 Dec 12. Autism Adulthood. 2023. PMID: 38116059 Free PMC article.
-
Trends in Surgical and Nonsurgical Aesthetic Procedures: A 14-Year Analysis of the International Society of Aesthetic Plastic Surgery-ISAPS.Aesthetic Plast Surg. 2024 Oct;48(20):4217-4227. doi: 10.1007/s00266-024-04260-2. Epub 2024 Aug 5. Aesthetic Plast Surg. 2024. PMID: 39103642 Review.
-
Australia in 2030: what is our path to health for all?Med J Aust. 2021 May;214 Suppl 8:S5-S40. doi: 10.5694/mja2.51020. Med J Aust. 2021. PMID: 33934362
Cited by
-
Recent advances in the biosynthesis of nucleoside antibiotics.J Antibiot (Tokyo). 2019 Dec;72(12):913-923. doi: 10.1038/s41429-019-0236-2. Epub 2019 Sep 25. J Antibiot (Tokyo). 2019. PMID: 31554958 Review.
-
C-C bond forming radical SAM enzymes involved in the construction of carbon skeletons of cofactors and natural products.Nat Prod Rep. 2018 Jul 18;35(7):660-694. doi: 10.1039/c8np00006a. Nat Prod Rep. 2018. PMID: 29633774 Free PMC article. Review.
-
An ATP-Dependent Ligase with Substrate Flexibility Involved in Assembly of the Peptidyl Nucleoside Antibiotic Polyoxin.Appl Environ Microbiol. 2018 Jun 18;84(13):e00501-18. doi: 10.1128/AEM.00501-18. Print 2018 Jul 1. Appl Environ Microbiol. 2018. PMID: 29703734 Free PMC article.
-
Radical Breakthroughs in Natural Product and Cofactor Biosynthesis.Biochemistry. 2018 Jan 30;57(4):390-402. doi: 10.1021/acs.biochem.7b00878. Epub 2017 Nov 9. Biochemistry. 2018. PMID: 29072833 Free PMC article. Review.
-
Biosynthesis and Genome Mining Potentials of Nucleoside Natural Products.Chembiochem. 2023 Sep 1;24(17):e202300342. doi: 10.1002/cbic.202300342. Epub 2023 Jul 26. Chembiochem. 2023. PMID: 37357819 Free PMC article.
References
-
- Isono K. J. Antibiot. 1988;41:1711. - PubMed
- Chen W., Qi J., Wu P., Wan D., Liu J., Feng X., Deng Z. J. Ind. Microbiol. Biotechnol. 2016;43:401. - PubMed
- Niu G., Tan H. Trends Microbiol. 2015;23:110. - PubMed
- Winn M., Goss R. J., Kimura K., Bugg T. D. Nat. Prod. Rep. 2010;27:279. - PubMed
- Lin C. I., McCarty R. M., Liu H. W. Chem. Soc. Rev. 2013;42:4377. - PMC - PubMed
-
- Isono K., Sato T., Hirasawa K., Funayama S., Suzuki S. J. Am. Chem. Soc. 1978;100:3937.
-
- Qi J., Liu J., Wan D., Cai Y. S., Wang Y., Li S., Wu P., Feng X., Qiu G., Yang S. P., Chen W., Deng Z. Biotechnol. Bioeng. 2015;112:1865. - PubMed
LinkOut - more resources
Full Text Sources
Other Literature Sources