Adenomatous polyposis coli (APC) protein moves along microtubules and concentrates at their growing ends in epithelial cells
- PMID: 10662776
- PMCID: PMC2174811
- DOI: 10.1083/jcb.148.3.505
Adenomatous polyposis coli (APC) protein moves along microtubules and concentrates at their growing ends in epithelial cells
Abstract
Adenomatous polyposis coli (APC) tumor suppressor protein has been shown to be localized near the distal ends of microtubules (MTs) at the edges of migrating cells. We expressed green fluorescent protein (GFP)-fusion proteins with full-length and deletion mutants of Xenopus APC in Xenopus epithelial cells, and observed their dynamic behavior in live cells. During cell spreading and wound healing, GFP-tagged full-length APC was concentrated as granules at the tip regions of cellular extensions. At higher magnification, APC appeared to move along MTs and concentrate as granules at the growing plus ends. When MTs began to shorten, the APC granules dropped off from the MT ends. Immunoelectron microscopy revealed that fuzzy structures surrounding MTs were the ultrastructural counterparts for these GFP signals. The COOH-terminal region of APC was targeted to the growing MT ends without forming granular aggregates, and abruptly disappeared when MTs began to shorten. The APC lacking the COOH-terminal region formed granular aggregates that moved along MTs toward their plus ends in an ATP-dependent manner. These findings indicated that APC is a unique MT-associated protein that moves along selected MTs and concentrates at their growing plus ends through their multiple functional domains.
Figures
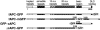
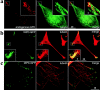
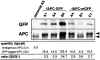
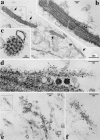
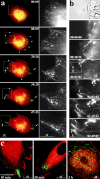
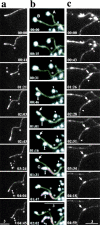
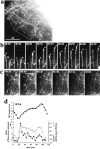
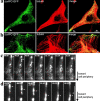
Similar articles
-
The dynamic behavior of the APC-binding protein EB1 on the distal ends of microtubules.Curr Biol. 2000 Jul 13;10(14):865-8. doi: 10.1016/s0960-9822(00)00600-x. Curr Biol. 2000. PMID: 10899006
-
Adenomatous polyposis coli (APC) protein regulates epithelial cell migration and morphogenesis via PDZ domain-based interactions with plasma membranes.Genes Cells. 2007 Feb;12(2):219-33. doi: 10.1111/j.1365-2443.2007.01045.x. Genes Cells. 2007. PMID: 17295841
-
Adenomatous polyposis coli on microtubule plus ends in cell extensions can promote microtubule net growth with or without EB1.Mol Biol Cell. 2006 May;17(5):2331-45. doi: 10.1091/mbc.e05-06-0498. Epub 2006 Mar 8. Mol Biol Cell. 2006. PMID: 16525027 Free PMC article.
-
The adenomatous polyposis coli protein: in the limelight out at the edge.Trends Cell Biol. 2001 Sep;11(9):378-84. doi: 10.1016/s0962-8924(01)02069-4. Trends Cell Biol. 2001. PMID: 11514192 Review.
-
Melanophores for microtubule dynamics and motility assays.Methods Cell Biol. 2010;97:401-14. doi: 10.1016/S0091-679X(10)97021-0. Methods Cell Biol. 2010. PMID: 20719282 Review.
Cited by
-
The role of histone methyltransferases in neurocognitive disorders associated with brain size abnormalities.Front Neurosci. 2023 Feb 10;17:989109. doi: 10.3389/fnins.2023.989109. eCollection 2023. Front Neurosci. 2023. PMID: 36845425 Free PMC article. Review.
-
Where is APC going?J Cell Biol. 2001 Sep 17;154(6):1105-9. doi: 10.1083/jcb.200106113. J Cell Biol. 2001. PMID: 11564752 Free PMC article. Review.
-
KIF17 stabilizes microtubules and contributes to epithelial morphogenesis by acting at MT plus ends with EB1 and APC.J Cell Biol. 2010 Aug 9;190(3):443-60. doi: 10.1083/jcb.201006044. J Cell Biol. 2010. PMID: 20696710 Free PMC article.
-
Drosophila EB1 is important for proper assembly, dynamics, and positioning of the mitotic spindle.J Cell Biol. 2002 Sep 2;158(5):873-84. doi: 10.1083/jcb.200202032. Epub 2002 Sep 3. J Cell Biol. 2002. PMID: 12213835 Free PMC article.
-
Apical membrane localization of the adenomatous polyposis coli tumor suppressor protein and subcellular distribution of the beta-catenin destruction complex in polarized epithelial cells.J Cell Biol. 2001 Feb 5;152(3):491-502. doi: 10.1083/jcb.152.3.491. J Cell Biol. 2001. PMID: 11157977 Free PMC article.
References
-
- Barth A.I., Näthke I.S., Nelson W.J. Cadherins, catenins and APC proteininterplay between cytoskeletal complexes and signaling pathways Curr. Opin. Cell Biol 9 1997. 683 690a - PubMed
-
- Ben-Ze'ev A., Geiger B. Differential molecular interactions of β-catenin and plakoglobin in adhesion, signaling and cancer. Curr. Opin. Cell Biol. 1998;10:629–639. - PubMed
MeSH terms
Substances
LinkOut - more resources
Full Text Sources
Other Literature Sources
Molecular Biology Databases