Changes in extracellular Ca2+ can affect the pattern of discharge in rat thalamic neurons
- PMID: 11507156
- PMCID: PMC2278774
- DOI: 10.1111/j.1469-7793.2001.00033.x
Changes in extracellular Ca2+ can affect the pattern of discharge in rat thalamic neurons
Abstract
1. The aim of this study was to investigate some of the cellular mechanisms involved in the effects caused by changes in extracellular Ca2+ concentration ([Ca2+](o)). 2. Current- and voltage-clamp experiments were carried out on acutely isolated thalamic neurons of rats. 3. Increasing [Ca2+](o) alone induced a transition of the discharge from single spike to burst mode in isolated current-clamped neurons. 4. Increasing [Ca(2+)](o) caused the voltage-dependent characteristics of the low voltage-activated (LVA) transient Ca2+ currents to shift towards positive values on the voltage axis. Changing [Ca2+](o) from 0.5 to 5 mM caused the inactivation curve to shift by 21 mV. 5. Extracellular Ca2+ blocked a steady cationic current. This current reversed at -35 mV, was scarcely affected by Mg2+ and was completely blocked by the non-selective cation channel inhibitor gadolinium (10 microM). The effect of [Ca2+](o) was mimicked by 500 microM spermine, a polyamine which acts as an agonist for the Ca(2+)-sensing receptor, and was modulated by intracellular GTP-gamma-S. 6. At the resting potential, both the voltage shift and the block of the inward current removed the inactivation of LVA calcium channels and, together with the increase in the Ca2+ driving force, favoured a rise in the low threshold Ca2+ spikes, causing the thalamic firing to change to the oscillatory mode. 7. Our data indicate that [Ca2+](o) is involved in multiple mechanisms of control of the thalamic relay and pacemaker activity. These findings shed light on the correlation between hypercalcaemia, low frequency EEG activity and symptoms such as sleepiness and lethargy described in many clinical papers.
Figures
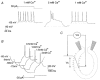
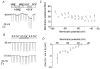
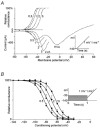
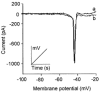
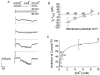
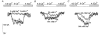
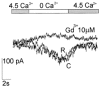
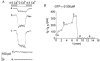
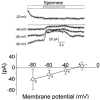
Similar articles
-
Calcium influx in rat thalamic relay neurons through voltage-dependent calcium channels is inhibited by enkephalin.Neurosci Lett. 1995 Dec 1;201(1):21-4. doi: 10.1016/0304-3940(95)12138-t. Neurosci Lett. 1995. PMID: 8830303
-
Modulation by extracellular pH of low- and high-voltage-activated calcium currents of rat thalamic relay neurons.J Neurophysiol. 2001 Mar;85(3):1051-8. doi: 10.1152/jn.2001.85.3.1051. J Neurophysiol. 2001. PMID: 11247975
-
Mechanisms for signal transformation in lemniscal auditory thalamus.J Neurophysiol. 1996 Dec;76(6):3597-608. doi: 10.1152/jn.1996.76.6.3597. J Neurophysiol. 1996. PMID: 8985860
-
Two distinct low-voltage-activated Ca2+ currents contribute to the pacemaker mechanism in cockroach dorsal unpaired median neurons.J Neurophysiol. 1996 Aug;76(2):963-76. doi: 10.1152/jn.1996.76.2.963. J Neurophysiol. 1996. PMID: 8871211
-
Effects of extracellular pH on voltage-gated Na+, K+ and Ca2+ currents in isolated rat CA1 neurons.J Physiol. 1996 Jun 15;493 ( Pt 3)(Pt 3):719-32. doi: 10.1113/jphysiol.1996.sp021417. J Physiol. 1996. PMID: 8799894 Free PMC article.
Cited by
-
Intellectual disability-associated UNC80 mutations reveal inter-subunit interaction and dendritic function of the NALCN channel complex.Nat Commun. 2020 Jul 3;11(1):3351. doi: 10.1038/s41467-020-17105-8. Nat Commun. 2020. PMID: 32620897 Free PMC article.
-
Hypocretins (orexins) regulate serotonin neurons in the dorsal raphe nucleus by excitatory direct and inhibitory indirect actions.J Neurosci. 2002 Nov 1;22(21):9453-64. doi: 10.1523/JNEUROSCI.22-21-09453.2002. J Neurosci. 2002. PMID: 12417670 Free PMC article.
-
TRPM7 channels in hippocampal neurons detect levels of extracellular divalent cations.Proc Natl Acad Sci U S A. 2007 Oct 9;104(41):16323-8. doi: 10.1073/pnas.0701149104. Epub 2007 Oct 3. Proc Natl Acad Sci U S A. 2007. PMID: 17913893 Free PMC article.
-
Novel vistas of calcium-mediated signalling in the thalamus.Pflugers Arch. 2004 May;448(2):131-8. doi: 10.1007/s00424-003-1234-5. Epub 2004 Feb 10. Pflugers Arch. 2004. PMID: 14770314 Review.
-
Genesis of interictal spikes in the CA1: a computational investigation.Front Neural Circuits. 2014 Jan 27;8:2. doi: 10.3389/fncir.2014.00002. eCollection 2014. Front Neural Circuits. 2014. PMID: 24478636 Free PMC article.
References
-
- Allen E M, Singer F R, Melamed D. Electroencephalographic abnormalities in hypercalcemia. Neurology. 1970;20:15–22. - PubMed
-
- Bragdon A C, Kojima H, Wilson W A. Suppression of interictal bursting in hippocampus unleashes seizures in entorhinal cortex: a proepileptic effect of lowering [K+] and raising [Ca2+] Brain Research. 1992;590:128–135. - PubMed
-
- Brown E M, Gamba G, Riccardi D, Lombardi M, Butters R, Kifor O, Sun A, Hediger M A, Lytton J, Hebert S C. Cloning and characterization of an extracellular Ca2+-sensing receptor from bovine parathyroid. Nature. 1993;366:575–580. - PubMed
MeSH terms
Substances
LinkOut - more resources
Full Text Sources
Miscellaneous