Altered axonal mitochondrial transport in the pathogenesis of Charcot-Marie-Tooth disease from mitofusin 2 mutations
- PMID: 17215403
- PMCID: PMC6672077
- DOI: 10.1523/JNEUROSCI.4798-06.2007
Altered axonal mitochondrial transport in the pathogenesis of Charcot-Marie-Tooth disease from mitofusin 2 mutations
Abstract
Mutations in the mitochondrial fusion protein mitofusin 2 (MFN2) are the most commonly identified cause of Charcot-Marie-Tooth type 2 (CMT2), a dominantly inherited disease characterized by degeneration of peripheral sensory and motor axons. However, the mechanism by which mutations in this ubiquitously expressed mitochondrial fusion protein lead to neuropathy has not yet been elucidated. To explore how MFN2 mutations lead to degeneration of peripheral axons, we expressed neuropathy-associated forms of MFN2 in cultured dorsal root ganglion neurons, cells preferentially affected in CMT2. Disease-associated MFN2 mutant proteins induced abnormal clustering of small fragmented mitochondria in both neuronal cell bodies and proximal axons. Interestingly, transport of mitochondria in axons was significantly impaired in neurons expressing disease-mutated forms of MFN2. The diminished axonal mitochondrial transport was not attributable to diminished ATP levels in the neurons, and oxidative respiration was normal in mutant MFN2-expressing cells. Additionally, mitochondrial oxidative enzyme activity was normal in muscle mitochondria from a CMT2 patient with an MFN2 mutation, further supporting that abnormal mitochondrial transport in neurons is independent from an energy production defect. This abnormal mitochondrial trafficking provides a likely explanation for the selective susceptibility of the longest peripheral axons to MFN2 mutations, in which proper localization of mitochondria is critical for axonal and synaptic function.
Figures
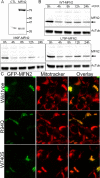
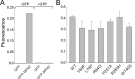
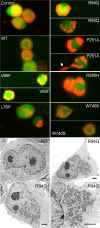
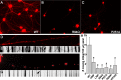
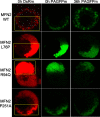
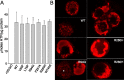
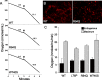
Similar articles
-
The Charcot-Marie Tooth Disease Mutation R94Q in MFN2 Decreases ATP Production but Increases Mitochondrial Respiration under Conditions of Mild Oxidative Stress.Cells. 2019 Oct 21;8(10):1289. doi: 10.3390/cells8101289. Cells. 2019. PMID: 31640251 Free PMC article.
-
Axonal Transport Defects in a Mitofusin 2 Loss of Function Model of Charcot-Marie-Tooth Disease in Zebrafish.PLoS One. 2013 Jun 26;8(6):e67276. doi: 10.1371/journal.pone.0067276. Print 2013. PLoS One. 2013. PMID: 23840650 Free PMC article.
-
Mitochondrial dynamics and inherited peripheral nerve diseases.Neurosci Lett. 2015 Jun 2;596:66-77. doi: 10.1016/j.neulet.2015.04.001. Epub 2015 Apr 3. Neurosci Lett. 2015. PMID: 25847151 Review.
-
Role of mitofusin 2 mutations in the physiopathology of Charcot-Marie-Tooth disease type 2A.Exp Neurol. 2009 Aug;218(2):268-73. doi: 10.1016/j.expneurol.2009.05.003. Epub 2009 May 8. Exp Neurol. 2009. PMID: 19427854 Review.
-
A human mitofusin 2 mutation can cause mitophagic cardiomyopathy.Elife. 2023 Nov 1;12:e84235. doi: 10.7554/eLife.84235. Elife. 2023. PMID: 37910431 Free PMC article.
Cited by
-
Microtubules Are Essential for Mitochondrial Dynamics-Fission, Fusion, and Motility-in Dictyostelium discoideum.Front Cell Dev Biol. 2016 Mar 22;4:19. doi: 10.3389/fcell.2016.00019. eCollection 2016. Front Cell Dev Biol. 2016. PMID: 27047941 Free PMC article.
-
Novel subcellular localization for α-synuclein: possible functional consequences.Front Neuroanat. 2015 Feb 23;9:17. doi: 10.3389/fnana.2015.00017. eCollection 2015. Front Neuroanat. 2015. PMID: 25755636 Free PMC article. Review.
-
Mitofusin 2 Deficiency Affects Energy Metabolism and Mitochondrial Biogenesis in MEF Cells.PLoS One. 2015 Jul 31;10(7):e0134162. doi: 10.1371/journal.pone.0134162. eCollection 2015. PLoS One. 2015. PMID: 26230519 Free PMC article.
-
Transporting mitochondria in neurons.F1000Res. 2016 Jul 18;5:F1000 Faculty Rev-1735. doi: 10.12688/f1000research.7864.1. eCollection 2016. F1000Res. 2016. PMID: 27508065 Free PMC article. Review.
-
Adaptor proteins MiD49 and MiD51 can act independently of Mff and Fis1 in Drp1 recruitment and are specific for mitochondrial fission.J Biol Chem. 2013 Sep 20;288(38):27584-27593. doi: 10.1074/jbc.M113.479873. Epub 2013 Aug 6. J Biol Chem. 2013. PMID: 23921378 Free PMC article.
References
-
- Araki T, Sasaki Y, Milbrandt J. Increased nuclear NAD biosynthesis and SIRT1 activation prevent axonal degeneration. Science. 2004;305:1010–1013. - PubMed
-
- Baloh RH, Tansey MG, Johnson EM, Jr, Milbrandt J. Functional mapping of receptor specificity domains of glial cell line- derived neurotrophic factor (GDNF) family ligands and production of GFRalpha1 RET-specific agonists. J Biol Chem. 2000;275:3412–3420. - PubMed
-
- Berger P, Young P, Suter U. Molecular cell biology of Charcot-Marie-Tooth disease. Neurogenetics. 2002;4:1–15. - PubMed
-
- Chada SR, Hollenbeck PJ. Mitochondrial movement and positioning in axons: the role of growth factor signaling. J Exp Biol. 2003;206:1985–1992. - PubMed
Publication types
MeSH terms
Substances
LinkOut - more resources
Full Text Sources
Other Literature Sources
Medical