Characterization of protein-protein interfaces
- PMID: 17851740
- PMCID: PMC2566606
- DOI: 10.1007/s10930-007-9108-x
Characterization of protein-protein interfaces
Abstract
We analyze the characteristics of protein-protein interfaces using the largest datasets available from the Protein Data Bank (PDB). We start with a comparison of interfaces with protein cores and non-interface surfaces. The results show that interfaces differ from protein cores and non-interface surfaces in residue composition, sequence entropy, and secondary structure. Since interfaces, protein cores, and non-interface surfaces have different solvent accessibilities, it is important to investigate whether the observed differences are due to the differences in solvent accessibility or differences in functionality. We separate out the effect of solvent accessibility by comparing interfaces with a set of residues having the same solvent accessibility as the interfaces. This strategy reveals residue distribution propensities that are not observable by comparing interfaces with protein cores and non-interface surfaces. Our conclusions are that there are larger numbers of hydrophobic residues, particularly aromatic residues, in interfaces, and the interactions apparently favored in interfaces include the opposite charge pairs and hydrophobic pairs. Surprisingly, Pro-Trp pairs are over represented in interfaces, presumably because of favorable geometries. The analysis is repeated using three datasets having different constraints on sequence similarity and structure quality. Consistent results are obtained across these datasets. We have also investigated separately the characteristics of heteromeric interfaces and homomeric interfaces.
Figures
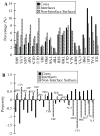
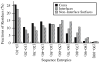
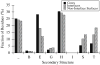
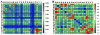
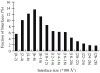
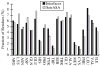
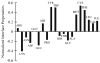
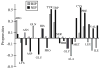
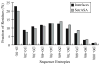
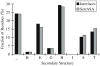
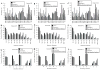
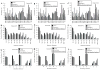
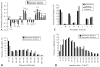
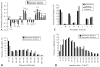
Similar articles
-
A comparative analysis of protein interfaces.Protein Pept Lett. 2010 Nov;17(11):1450-8. doi: 10.2174/0929866511009011450. Protein Pept Lett. 2010. PMID: 20666728
-
A PDB-wide, evolution-based assessment of protein-protein interfaces.BMC Struct Biol. 2014 Oct 18;14:22. doi: 10.1186/s12900-014-0022-0. BMC Struct Biol. 2014. PMID: 25326082 Free PMC article.
-
Residue frequencies and pairing preferences at protein-protein interfaces.Proteins. 2001 May 1;43(2):89-102. Proteins. 2001. PMID: 11276079
-
Protein-protein interfaces: architectures and interactions in protein-protein interfaces and in protein cores. Their similarities and differences.Crit Rev Biochem Mol Biol. 1996 Apr;31(2):127-52. doi: 10.3109/10409239609106582. Crit Rev Biochem Mol Biol. 1996. PMID: 8740525 Review.
-
Geometry of nonbonded interactions involving planar groups in proteins.Prog Biophys Mol Biol. 2007 Sep-Nov;95(1-3):83-137. doi: 10.1016/j.pbiomolbio.2007.03.016. Epub 2007 Jun 5. Prog Biophys Mol Biol. 2007. PMID: 17629549 Review.
Cited by
-
Assessment of protein-protein interfaces in cryo-EM derived assemblies.Nat Commun. 2021 Jun 7;12(1):3399. doi: 10.1038/s41467-021-23692-x. Nat Commun. 2021. PMID: 34099703 Free PMC article.
-
Phosphorylation promotes binding affinity of Rap-Raf complex by allosteric modulation of switch loop dynamics.Sci Rep. 2018 Aug 28;8(1):12976. doi: 10.1038/s41598-018-31234-7. Sci Rep. 2018. PMID: 30154518 Free PMC article.
-
A proactive role of water molecules in acceptor recognition by protein O-fucosyltransferase 2.Nat Chem Biol. 2016 Apr;12(4):240-6. doi: 10.1038/nchembio.2019. Epub 2016 Feb 8. Nat Chem Biol. 2016. PMID: 26854667 Free PMC article.
-
Multiple Domain Associations within the Arabidopsis Immune Receptor RPP1 Regulate the Activation of Programmed Cell Death.PLoS Pathog. 2016 Jul 18;12(7):e1005769. doi: 10.1371/journal.ppat.1005769. eCollection 2016 Jul. PLoS Pathog. 2016. PMID: 27427964 Free PMC article.
-
Evolution of In Silico Strategies for Protein-Protein Interaction Drug Discovery.Molecules. 2018 Aug 6;23(8):1963. doi: 10.3390/molecules23081963. Molecules. 2018. PMID: 30082644 Free PMC article. Review.
References
-
- Chothia C, Janin J. Nature. 1975;256:705–708. - PubMed
-
- Wodak SJ, Janin J. Adv Protein Chem. 2002;61:9–73. - PubMed
-
- Deremble C, Lavery R. Curr Opin Struct Biol. 2005;15:171–175. - PubMed
-
- Ponstingl H, Kabir T, Gorse D, Thornton JM. Progr Bio-phys Mol Biol. 2005;89:9–35. - PubMed
-
- Reichmann D, Rahat O, Cohen M, Neuvirth H, Schreiber G. Curr Opin Struct Biol. 2007;17:67–76. - PubMed
Publication types
MeSH terms
Substances
Grants and funding
LinkOut - more resources
Full Text Sources
Other Literature Sources