Reptile scale paradigm: Evo-Devo, pattern formation and regeneration
- PMID: 19557687
- PMCID: PMC2874329
- DOI: 10.1387/ijdb.072556cc
Reptile scale paradigm: Evo-Devo, pattern formation and regeneration
Abstract
The purpose of this perspective is to highlight the merit of the reptile integument as an experimental model. Reptiles represent the first amniotes. From stem reptiles, extant reptiles, birds and mammals have evolved. Mammal hairs and feathers evolved from Therapsid and Sauropsid reptiles, respectively. The early reptilian integument had to adapt to the challenges of terrestrial life, developing a multi-layered stratum corneum capable of barrier function and ultraviolet protection. For better mechanical protection, diverse reptilian scale types have evolved. The evolution of endothermy has driven the convergent evolution of hair and feather follicles: both form multiple localized growth units with stem cells and transient amplifying cells protected in the proximal follicle. This topological arrangement allows them to elongate, molt and regenerate without structural constraints. Another unique feature of reptile skin is the exquisite arrangement of scales and pigment patterns, making them testable models for mechanisms of pattern formation. Since they face the constant threat of damage on land, different strategies were developed to accommodate skin homeostasis and regeneration. Temporally, they can be under continuous renewal or sloughing cycles. Spatially, they can be diffuse or form discrete localized growth units (follicles). To understand how gene regulatory networks evolved to produce increasingly complex ectodermal organs, we have to study how prototypic scale-forming pathways in reptiles are modulated to produce appendage novelties. Despite the fact that there are numerous studies of reptile scales, molecular analyses have lagged behind. Here, we underscore how further development of this novel experimental model will be valuable in filling the gaps of our understanding of the Evo-Devo of amniote integuments.
Figures
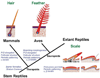
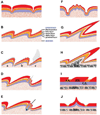
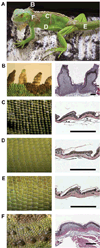
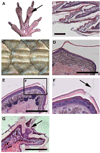
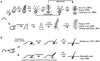
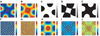
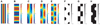
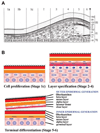
Comment in
-
Pattern formation today.Int J Dev Biol. 2009;53(5-6):653-8. doi: 10.1387/ijdb.082594cc. Int J Dev Biol. 2009. PMID: 19557673 Free PMC article. Review.
Similar articles
-
Evo-Devo of amniote integuments and appendages.Int J Dev Biol. 2004;48(2-3):249-70. doi: 10.1387/ijdb.041825pw. Int J Dev Biol. 2004. PMID: 15272390 Free PMC article. Review.
-
Adaptation to the land: The skin of reptiles in comparison to that of amphibians and endotherm amniotes.J Exp Zool B Mol Dev Evol. 2003 Aug 15;298(1):12-41. doi: 10.1002/jez.b.24. J Exp Zool B Mol Dev Evol. 2003. PMID: 12949767 Review.
-
Dermo-epidermal interactions in reptilian scales: speculations on the evolution of scales, feathers, and hairs.J Exp Zool B Mol Dev Evol. 2004 Jul 15;302(4):365-83. doi: 10.1002/jez.b.20028. J Exp Zool B Mol Dev Evol. 2004. PMID: 15287101
-
A new scenario for the evolutionary origin of hair, feather, and avian scales.J Anat. 2009 Apr;214(4):587-606. doi: 10.1111/j.1469-7580.2008.01041.x. J Anat. 2009. PMID: 19422430 Free PMC article. Review.
-
The anatomical placode in reptile scale morphogenesis indicates shared ancestry among skin appendages in amniotes.Sci Adv. 2016 Jun 24;2(6):e1600708. doi: 10.1126/sciadv.1600708. eCollection 2016 Jun. Sci Adv. 2016. PMID: 28439533 Free PMC article.
Cited by
-
Pathology of "double scale" skin defect in farmed American alligators (Alligator mississippiensis) and the possible association with hepatic fibrosis.Vet Pathol. 2024 Sep;61(5):815-828. doi: 10.1177/03009858241238685. Epub 2024 Mar 29. Vet Pathol. 2024. PMID: 38549443 Free PMC article.
-
Ultrastructural evidence of a mechanosensory function of scale organs (sensilla) in sea snakes (Hydrophiinae).R Soc Open Sci. 2019 Apr 10;6(4):182022. doi: 10.1098/rsos.182022. eCollection 2019 Apr. R Soc Open Sci. 2019. PMID: 31183131 Free PMC article.
-
Molecular and Cellular Characterization of Avian Reticulate Scales Implies the Evo-Devo Novelty of Skin Appendages in Foot Sole.J Dev Biol. 2023 Jul 3;11(3):30. doi: 10.3390/jdb11030030. J Dev Biol. 2023. PMID: 37489331 Free PMC article.
-
Seasonal changes in steroid and thyroid hormone content in shed skins of the tegu lizard Salvator merianae.J Comp Physiol B. 2022 Jan;192(1):127-139. doi: 10.1007/s00360-021-01397-3. Epub 2021 Aug 11. J Comp Physiol B. 2022. PMID: 34379176
-
Crocodylians evolved scattered multi-sensory micro-organs.Evodevo. 2013 Jul 2;4(1):19. doi: 10.1186/2041-9139-4-19. Evodevo. 2013. PMID: 23819918 Free PMC article.
References
-
- Alexander NJ. Comparison of α an β keratin in reptiles. Z. Zellforsch. 1970;110:153–165. - PubMed
-
- Alibardi L. Keratohyalin-like granules and differentiation of the shedding complex in embryonic and regenerating epidermis of lizards and Sphenodon (Reptilia, Lepidosauria) Amphibia-Reptilia. 1999;20:11–23.
-
- Alibardi L. Ultrastructural localization of alpha-keratins in the regenerating epidermis of the lizard Podarcis muralis during formation of the shedding layer. Tissue Cell. 2000;32:153–162. - PubMed
Publication types
MeSH terms
Grants and funding
LinkOut - more resources
Full Text Sources
Other Literature Sources