Biophysics of magnetic orientation: strengthening the interface between theory and experimental design
- PMID: 20071390
- PMCID: PMC2843999
- DOI: 10.1098/rsif.2009.0491.focus
Biophysics of magnetic orientation: strengthening the interface between theory and experimental design
Abstract
The first demonstrations of magnetic effects on the behaviour of migratory birds and homing pigeons in laboratory and field experiments, respectively, provided evidence for the longstanding hypothesis that animals such as birds that migrate and home over long distances would benefit from possession of a magnetic sense. Subsequent identification of at least two plausible biophysical mechanisms for magnetoreception in animals, one based on biogenic magnetite and another on radical-pair biochemical reactions, led to major efforts over recent decades to test predictions of the two models, as well as efforts to understand the ultrastructure and function of the possible magnetoreceptor cells. Unfortunately, progress in understanding the magnetic sense has been challenged by: (i) the availability of a relatively small number of techniques for analysing behavioural responses to magnetic fields by animals; (ii) difficulty in achieving reproducible results using the techniques; and (iii) difficulty in development and implementation of new techniques that might bring greater experimental power. As a consequence, laboratory and field techniques used to study the magnetic sense today remain substantially unchanged, despite the huge developments in technology and instrumentation since the techniques were developed in the 1950s. New methods developed for behavioural study of the magnetic sense over the last 30 years include the use of laboratory conditioning techniques and tracking devices based on transmission of radio signals to and from satellites. Here we consider methodological developments in the study of the magnetic sense and present suggestions for increasing the reproducibility and ease of interpretation of experimental studies. We recommend that future experiments invest more effort in automating control of experiments and data capture, control of stimulation and full blinding of experiments in the rare cases where automation is impossible. We also propose new experiments to confirm whether or not animals can detect magnetic fields using the radical-pair effect together with an alternate hypothesis that may explain the dependence on light of responses by animals to magnetic field stimuli.
Figures
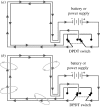
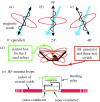
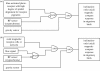
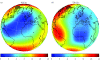
Similar articles
-
Cryptochromes--a potential magnetoreceptor: what do we know and what do we want to know?J R Soc Interface. 2010 Apr 6;7 Suppl 2(Suppl 2):S147-62. doi: 10.1098/rsif.2009.0411.focus. Epub 2009 Nov 11. J R Soc Interface. 2010. PMID: 19906675 Free PMC article.
-
Avian magnetite-based magnetoreception: a physiologist's perspective.J R Soc Interface. 2010 Apr 6;7 Suppl 2(Suppl 2):S193-205. doi: 10.1098/rsif.2009.0423.focus. Epub 2010 Jan 27. J R Soc Interface. 2010. PMID: 20106875 Free PMC article.
-
Directional orientation of birds by the magnetic field under different light conditions.J R Soc Interface. 2010 Apr 6;7 Suppl 2(Suppl 2):S163-77. doi: 10.1098/rsif.2009.0367.focus. Epub 2009 Oct 28. J R Soc Interface. 2010. PMID: 19864263 Free PMC article. Review.
-
The puzzle of magnetic resonance effect on the magnetic compass of migratory birds.Bioelectromagnetics. 2009 Jul;30(5):402-10. doi: 10.1002/bem.20485. Bioelectromagnetics. 2009. PMID: 19291711
-
Magnetoreception in birds.J R Soc Interface. 2019 Sep 27;16(158):20190295. doi: 10.1098/rsif.2019.0295. Epub 2019 Sep 4. J R Soc Interface. 2019. PMID: 31480921 Free PMC article. Review.
Cited by
-
Physical limits to magnetogenetics.Elife. 2016 Aug 16;5:e17210. doi: 10.7554/eLife.17210. Elife. 2016. PMID: 27529126 Free PMC article.
-
Integration and evaluation of magnetic stimulation in physiology setups.PLoS One. 2022 Jul 22;17(7):e0271765. doi: 10.1371/journal.pone.0271765. eCollection 2022. PLoS One. 2022. PMID: 35867646 Free PMC article.
-
Magnetic characterization of isolated candidate vertebrate magnetoreceptor cells.Proc Natl Acad Sci U S A. 2012 Jul 24;109(30):12022-7. doi: 10.1073/pnas.1205653109. Epub 2012 Jul 9. Proc Natl Acad Sci U S A. 2012. PMID: 22778440 Free PMC article.
-
Bringing the analysis of animal orientation data full circle: model-based approaches with maximum likelihood.J Exp Biol. 2017 Nov 1;220(Pt 21):3878-3882. doi: 10.1242/jeb.167056. Epub 2017 Aug 31. J Exp Biol. 2017. PMID: 28860118 Free PMC article.
-
Avian magnetic compass can be tuned to anomalously low magnetic intensities.Proc Biol Sci. 2013 May 29;280(1763):20130853. doi: 10.1098/rspb.2013.0853. Print 2013 Jul 22. Proc Biol Sci. 2013. PMID: 23720547 Free PMC article.
References
-
- Bazylinski D. A., Schlezinger D. R., Howes B. H., Frankel R. B., Epstein S. S. 2000. Occurrence and distribution of diverse populations of magnetic protists in a chemically stratified coastal salt pond. Chem. Geol. 169, 319–328. (10.1016/S0009-2541(00)00211-4) - DOI
-
- Beason R. C., Wiltschko R., Wiltschko W. 1997. Pigeon homing: effects of magnetic pulses on initial orientation. Auk 114, 405–415.
-
- Beaugrand J. P. 1976. An attempt to confirm magnetic sensitivity in the pigeon, Columba livia. J. Comp. Phys. 110, 343–355. (10.1007/BF00659150) - DOI
Publication types
MeSH terms
Grants and funding
LinkOut - more resources
Full Text Sources
Research Materials