The mechanism of prion inhibition by HET-S
- PMID: 20620958
- PMCID: PMC3507513
- DOI: 10.1016/j.molcel.2010.05.019
The mechanism of prion inhibition by HET-S
Abstract
HET-S (97% identical to HET-s) has an N-terminal globular domain that exerts a prion-inhibitory effect in cis on its own prion-forming domain (PFD) and in trans on HET-s prion propagation. We show that HET-S fails to form fibrils in vitro and that it inhibits HET-s PFD fibrillization in trans. In vivo analyses indicate that beta-structuring of the HET-S PFD is required for HET-S activity. The crystal structures of the globular domains of HET-s and HET-S are highly similar, comprising a helical fold, while NMR-based characterizations revealed no differences in the conformations of the PFDs. We conclude that prion inhibition is not encoded by structure but rather in stability and oligomerization properties: when HET-S forms a prion seed or is incorporated into a HET-s fibril via its PFD, the beta-structuring in this domain induces a change in its globular domain, generating a molecular species that is incompetent for fibril growth.
Copyright (c) 2010 Elsevier Inc. All rights reserved.
Figures
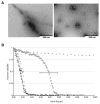
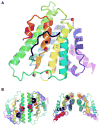
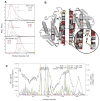
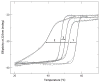
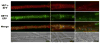
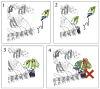
Similar articles
-
Genomic clustering and homology between HET-S and the NWD2 STAND protein in various fungal genomes.PLoS One. 2012;7(4):e34854. doi: 10.1371/journal.pone.0034854. Epub 2012 Apr 6. PLoS One. 2012. PMID: 22493719 Free PMC article.
-
Contribution of specific residues of the β-solenoid fold to HET-s prion function, amyloid structure and stability.PLoS Pathog. 2014 Jun 12;10(6):e1004158. doi: 10.1371/journal.ppat.1004158. eCollection 2014 Jun. PLoS Pathog. 2014. PMID: 24945274 Free PMC article.
-
The [Het-s] prion of Podospora anserina and its role in heterokaryon incompatibility.Semin Cell Dev Biol. 2011 Jul;22(5):460-8. doi: 10.1016/j.semcdb.2011.02.019. Epub 2011 Feb 18. Semin Cell Dev Biol. 2011. PMID: 21334447 Review.
-
Signal transduction by a fungal NOD-like receptor based on propagation of a prion amyloid fold.PLoS Biol. 2015 Feb 11;13(2):e1002059. doi: 10.1371/journal.pbio.1002059. eCollection 2015 Feb. PLoS Biol. 2015. PMID: 25671553 Free PMC article.
-
Structure, function, and amyloidogenesis of fungal prions: filament polymorphism and prion variants.Adv Protein Chem. 2006;73:125-80. doi: 10.1016/S0065-3233(06)73005-4. Adv Protein Chem. 2006. PMID: 17190613 Review.
Cited by
-
Key Points Concerning Amyloid Infectivity and Prion-Like Neuronal Invasion.Front Mol Neurosci. 2016 Apr 22;9:29. doi: 10.3389/fnmol.2016.00029. eCollection 2016. Front Mol Neurosci. 2016. PMID: 27147962 Free PMC article.
-
High natural prevalence of a fungal prion.Proc Natl Acad Sci U S A. 2012 Jun 26;109(26):10432-7. doi: 10.1073/pnas.1205333109. Epub 2012 Jun 12. Proc Natl Acad Sci U S A. 2012. PMID: 22691498 Free PMC article.
-
Heterogeneous seeding of HET-s(218-289) and the mutability of prion structures.Prion. 2014 Mar-Apr;8(2):178-82. doi: 10.4161/pri.28126. Epub 2014 Feb 18. Prion. 2014. PMID: 24549096 Free PMC article. Review.
-
Amyloid by Design: Intrinsic Regulation of Microbial Amyloid Assembly.J Mol Biol. 2018 Oct 12;430(20):3631-3641. doi: 10.1016/j.jmb.2018.07.007. Epub 2018 Jul 12. J Mol Biol. 2018. PMID: 30017921 Free PMC article. Review.
-
The expanding scope of amyloid signalling.Prion. 2021 Dec;15(1):21-28. doi: 10.1080/19336896.2021.1874791. Prion. 2021. PMID: 33573441 Free PMC article.
References
-
- Aguzzi A, Sigurdson C, Heikenwaelder M. Molecular mechanisms of prion pathogenesis. Annu Rev Pathol. 2008;3:11–40. - PubMed
-
- Balguerie A, Dos Reis S, Coulary-Salin B, Chaignepain S, Sabourin M, Schmitter JM, Saupe SJ. The sequences appended to the amyloid core region of the HET-s prion protein determine higher-order aggregate organization in vivo. J Cell Sci. 2004;117:2599–2610. - PubMed
-
- Beisson-Schecroun J. Incompatibilité cellulaire et interactions nucléocytoplamsiques dans les phénoménes de barrage chez le Podospora anserina. Ann Genet. 1962;4:3–50. - PubMed
Publication types
MeSH terms
Substances
Grants and funding
LinkOut - more resources
Full Text Sources