Influence of microbial biofilms on the preservation of primary soft tissue in fossil and extant archosaurs
- PMID: 20967227
- PMCID: PMC2953520
- DOI: 10.1371/journal.pone.0013334
Influence of microbial biofilms on the preservation of primary soft tissue in fossil and extant archosaurs
Abstract
Background: Mineralized and permineralized bone is the most common form of fossilization in the vertebrate record. Preservation of gross soft tissues is extremely rare, but recent studies have suggested that primary soft tissues and biomolecules are more commonly preserved within preserved bones than had been presumed. Some of these claims have been challenged, with presentation of evidence suggesting that some of the structures are microbial artifacts, not primary soft tissues. The identification of biomolecules in fossil vertebrate extracts from a specimen of Brachylophosaurus canadensis has shown the interpretation of preserved organic remains as microbial biofilm to be highly unlikely. These discussions also propose a variety of potential mechanisms that would permit the preservation of soft-tissues in vertebrate fossils over geologic time.
Methodology/principal findings: This study experimentally examines the role of microbial biofilms in soft-tissue preservation in vertebrate fossils by quantitatively establishing the growth and morphology of biofilms on extant archosaur bone. These results are microscopically and morphologically compared with soft-tissue extracts from vertebrate fossils from the Hell Creek Formation of southeastern Montana (Latest Maastrichtian) in order to investigate the potential role of microbial biofilms on the preservation of fossil bone and bound organic matter in a variety of taphonomic settings. Based on these analyses, we highlight a mechanism whereby this bound organic matter may be preserved.
Conclusions/significance: Results of the study indicate that the crystallization of microbial biofilms on decomposing organic matter within vertebrate bone in early taphonomic stages may contribute to the preservation of primary soft tissues deeper in the bone structure.
Conflict of interest statement
Figures
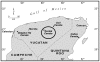
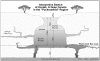
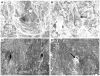
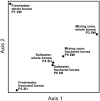
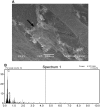
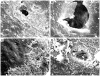
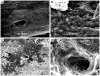
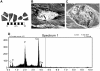
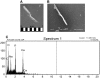
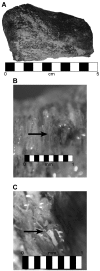
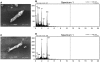
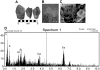
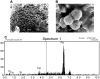
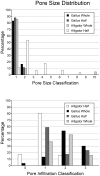
Similar articles
-
Cretaceous dinosaur bone contains recent organic material and provides an environment conducive to microbial communities.Elife. 2019 Jun 18;8:e46205. doi: 10.7554/eLife.46205. Elife. 2019. PMID: 31210129 Free PMC article.
-
Embryo fossilization is a biological process mediated by microbial biofilms.Proc Natl Acad Sci U S A. 2008 Dec 9;105(49):19360-5. doi: 10.1073/pnas.0810106105. Epub 2008 Dec 1. Proc Natl Acad Sci U S A. 2008. PMID: 19047625 Free PMC article.
-
The Effect Of microbial Mats In The Decay Of Anurans With Implications For Understanding Taphonomic Processes In The Fossil Record.Sci Rep. 2017 Mar 24;7:45160. doi: 10.1038/srep45160. Sci Rep. 2017. PMID: 28338095 Free PMC article.
-
Soft-Bodied Fossils Are Not Simply Rotten Carcasses - Toward a Holistic Understanding of Exceptional Fossil Preservation: Exceptional Fossil Preservation Is Complex and Involves the Interplay of Numerous Biological and Geological Processes.Bioessays. 2018 Jan;40(1). doi: 10.1002/bies.201700167. Epub 2017 Nov 29. Bioessays. 2018. PMID: 29193177 Review.
-
Hunting the Snark: the flawed search for mythical Jurassic angiosperms.J Exp Bot. 2020 Jan 1;71(1):22-35. doi: 10.1093/jxb/erz411. J Exp Bot. 2020. PMID: 31538196 Review.
Cited by
-
Report of bioerosions and cells in Cainotheriidae (Mammalia, Artiodactyla) from the phosphorites of Quercy (SW France).Sci Rep. 2024 Oct 10;14(1):23708. doi: 10.1038/s41598-024-74301-y. Sci Rep. 2024. PMID: 39390074 Free PMC article.
-
Genome-centric resolution of novel microbial lineages in an excavated Centrosaurus dinosaur fossil bone from the Late Cretaceous of North America.Environ Microbiome. 2020 Mar 19;15(1):8. doi: 10.1186/s40793-020-00355-w. Environ Microbiome. 2020. PMID: 33902738 Free PMC article.
-
Microbially induced calcium carbonate precipitation in fossil consolidation treatments: Preliminary results inducing exogenous Myxococcus xanthus bacteria in a miocene Cheirogaster richardi specimen.Heliyon. 2023 Jun 22;9(7):e17597. doi: 10.1016/j.heliyon.2023.e17597. eCollection 2023 Jul. Heliyon. 2023. PMID: 37449105 Free PMC article.
-
Microspectroscopic evidence of cretaceous bone proteins.PLoS One. 2011 Apr 29;6(4):e19445. doi: 10.1371/journal.pone.0019445. PLoS One. 2011. PMID: 21559386 Free PMC article.
-
Deciphering pyritization-kerogenization gradient for fish soft-tissue preservation.Sci Rep. 2017 May 3;7(1):1468. doi: 10.1038/s41598-017-01563-0. Sci Rep. 2017. PMID: 28469235 Free PMC article.
References
-
- Schweitzer MH, Wittmeyer JL, Horner JR, Toporski JK. Soft-tissue vessels and cellular preservation in Tyrannosaurus rex. Science. 2005;307:1952–1955. - PubMed
-
- Asara JM, Schweitzer MH, Freimark LM, Philips M, Cantley LC. Protein sequences from Mastodon and Tyrannosaurus rex revealed by mass spectrometry. Science. 2007;31:280–285. - PubMed
-
- Schweitzer MH, Zheng W, Organ CL, Avci R, Suo Z, et al. Biomolecular characterization and protein sequences of the Campanian Hadrosaur B. canadensis. Science. 2009;324:626–631. - PubMed
-
- Kaye TG, Gaugler G, Sawlowicz Z. Dinosaurian soft tissue interpreted as bacterial biofilms. PloS ONE. 2008;3(7):e2808. doi: 10.1371/journal.pone.0002808. - DOI - PMC - PubMed
-
- Dodson P. The significance of small bones in paleoecological interpretations. University of Wyoming Contributions to Geology. 1973;12:15–19.
Publication types
MeSH terms
LinkOut - more resources
Full Text Sources