Equiluminance cells in visual cortical area v4
- PMID: 21880901
- PMCID: PMC3171995
- DOI: 10.1523/JNEUROSCI.1890-11.2011
Equiluminance cells in visual cortical area v4
Abstract
We report a novel class of V4 neuron in the macaque monkey that responds selectively to equiluminant colored form. These "equiluminance" cells stand apart because they violate the well established trend throughout the visual system that responses are minimal at low luminance contrast and grow and saturate as contrast increases. Equiluminance cells, which compose ∼22% of V4, exhibit the opposite behavior: responses are greatest near zero contrast and decrease as contrast increases. While equiluminance cells respond preferentially to equiluminant colored stimuli, strong hue tuning is not their distinguishing feature-some equiluminance cells do exhibit strong unimodal hue tuning, but many show little or no tuning for hue. We find that equiluminance cells are color and shape selective to a degree comparable with other classes of V4 cells with more conventional contrast response functions. Those more conventional cells respond equally well to achromatic luminance and equiluminant color stimuli, analogous to color luminance cells described in V1. The existence of equiluminance cells, which have not been reported in V1 or V2, suggests that chromatically defined boundaries and shapes are given special status in V4 and raises the possibility that form at equiluminance and form at higher contrasts are processed in separate channels in V4.
Figures
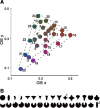
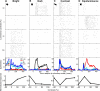
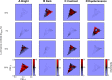
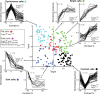
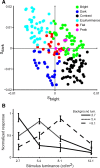
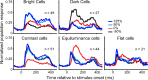
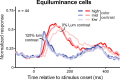
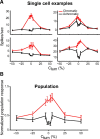
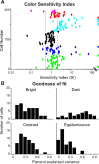
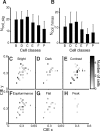
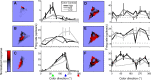
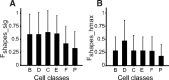
Similar articles
-
Neuronal mechanisms of color categorization in areas V1, V2 and V4 of macaque monkey visual cortex.Behav Brain Res. 1996 Apr;76(1-2):51-70. doi: 10.1016/0166-4328(95)00183-2. Behav Brain Res. 1996. PMID: 8734043
-
Hue and orientation pinwheels in macaque area V4.J Neurophysiol. 2024 Aug 1;132(2):589-615. doi: 10.1152/jn.00366.2023. Epub 2024 Jul 11. J Neurophysiol. 2024. PMID: 38988289
-
Search for color 'center(s)' in macaque visual cortex.Cereb Cortex. 2004 Apr;14(4):353-63. doi: 10.1093/cercor/bhh001. Cereb Cortex. 2004. PMID: 15028640
-
Equiluminant red-green and blue-yellow VEPs in multiple sclerosis.J Clin Neurophysiol. 2001 Nov;18(6):583-91. doi: 10.1097/00004691-200111000-00010. J Clin Neurophysiol. 2001. PMID: 11779973
-
Neural mechanisms for color perception in the primary visual cortex.Curr Opin Neurobiol. 2002 Aug;12(4):426-32. doi: 10.1016/s0959-4388(02)00349-5. Curr Opin Neurobiol. 2002. PMID: 12139991 Review.
Cited by
-
The Attentional Suppressive Surround: Eccentricity, Location-Based and Feature-Based Effects and Interactions.Front Neurosci. 2018 Oct 8;12:710. doi: 10.3389/fnins.2018.00710. eCollection 2018. Front Neurosci. 2018. PMID: 30349452 Free PMC article.
-
Shape encoding consistency across colors in primate V4.J Neurophysiol. 2012 Sep;108(5):1299-308. doi: 10.1152/jn.01063.2011. Epub 2012 Jun 6. J Neurophysiol. 2012. PMID: 22673324 Free PMC article.
-
A cortical edge-integration model of object-based lightness computation that explains effects of spatial context and individual differences.Front Hum Neurosci. 2014 Aug 22;8:640. doi: 10.3389/fnhum.2014.00640. eCollection 2014. Front Hum Neurosci. 2014. PMID: 25202253 Free PMC article.
-
Comparative connectomics reveals noncanonical wiring for color vision in human foveal retina.Proc Natl Acad Sci U S A. 2023 May 2;120(18):e2300545120. doi: 10.1073/pnas.2300545120. Epub 2023 Apr 25. Proc Natl Acad Sci U S A. 2023. PMID: 37098066 Free PMC article.
-
Neural correlates of dynamic lightness induction.J Vis. 2024 Sep 3;24(9):10. doi: 10.1167/jov.24.9.10. J Vis. 2024. PMID: 39259170 Free PMC article.
References
-
- Albrecht DG. Visual cortex neurons in monkey and cat: effect of contrast on the spatial and temporal phase transfer functions. Vis Neurosci. 1995;12:1191–1210. - PubMed
-
- Bichot NP, Rossi AF, Desimone R. Parallel and serial neural mechanisms for visual search in macaque area V4. Science. 2005;308:529–534. - PubMed
-
- Bradley A, Switkes E, De Valois K. Orientation and spatial frequency selectivity of adaptation to color and luminance gratings. Vision Res. 1988;28:841–856. - PubMed
Publication types
MeSH terms
Grants and funding
LinkOut - more resources
Full Text Sources