Mechanosensitive channels: what can they do and how do they do it?
- PMID: 22000509
- PMCID: PMC3203646
- DOI: 10.1016/j.str.2011.09.005
Mechanosensitive channels: what can they do and how do they do it?
Abstract
While mechanobiological processes employ diverse mechanisms, at their heart are force-induced perturbations in the structure and dynamics of molecules capable of triggering subsequent events. Among the best characterized force-sensing systems are bacterial mechanosensitive channels. These channels reflect an intimate coupling of protein conformation with the mechanics of the surrounding membrane; the membrane serves as an adaptable sensor that responds to an input of applied force and converts it into an output signal, interpreted for the cell by mechanosensitive channels. The cell can exploit this information in a number of ways: ensuring cellular viability in the presence of osmotic stress and perhaps also serving as a signal transducer for membrane tension or other functions. This review focuses on the bacterial mechanosensitive channels of large (MscL) and small (MscS) conductance and their eukaryotic homologs, with an emphasis on the outstanding issues surrounding the function and mechanism of this fascinating class of molecules.
Copyright © 2011 Elsevier Ltd. All rights reserved.
Figures
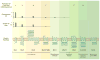
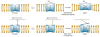
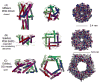
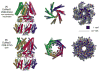
Similar articles
-
Structural mechanism for gating of a eukaryotic mechanosensitive channel of small conductance.Nat Commun. 2020 Jul 23;11(1):3690. doi: 10.1038/s41467-020-17538-1. Nat Commun. 2020. PMID: 32704140 Free PMC article.
-
The role of MscL amphipathic N terminus indicates a blueprint for bilayer-mediated gating of mechanosensitive channels.Nat Commun. 2016 Jun 22;7:11984. doi: 10.1038/ncomms11984. Nat Commun. 2016. PMID: 27329693 Free PMC article.
-
MscS-like mechanosensitive channels in plants and microbes.Biochemistry. 2013 Aug 27;52(34):5708-22. doi: 10.1021/bi400804z. Epub 2013 Aug 15. Biochemistry. 2013. PMID: 23947546 Free PMC article. Review.
-
Characterizing the mechanosensitive response of Paraburkholderia graminis membranes.Biochim Biophys Acta Biomembr. 2020 Apr 1;1862(4):183176. doi: 10.1016/j.bbamem.2020.183176. Epub 2020 Jan 7. Biochim Biophys Acta Biomembr. 2020. PMID: 31923411
-
Gating the bacterial mechanosensitive channels: MscS a new paradigm?Curr Opin Microbiol. 2004 Apr;7(2):163-7. doi: 10.1016/j.mib.2004.02.006. Curr Opin Microbiol. 2004. PMID: 15063854 Review.
Cited by
-
Structural mechanism for gating of a eukaryotic mechanosensitive channel of small conductance.Nat Commun. 2020 Jul 23;11(1):3690. doi: 10.1038/s41467-020-17538-1. Nat Commun. 2020. PMID: 32704140 Free PMC article.
-
Mechanical systems biology of C. elegans touch sensation.Bioessays. 2015 Mar;37(3):335-44. doi: 10.1002/bies.201400154. Epub 2015 Jan 19. Bioessays. 2015. PMID: 25597279 Free PMC article. Review.
-
How Functional Lipids Affect the Structure and Gating of Mechanosensitive MscS-like Channels.Int J Mol Sci. 2022 Dec 1;23(23):15071. doi: 10.3390/ijms232315071. Int J Mol Sci. 2022. PMID: 36499396 Free PMC article. Review.
-
Characterization of the genome from Geobacter anodireducens, a strain with enhanced current production in bioelectrochemical systems.RSC Adv. 2019 Aug 19;9(44):25890-25899. doi: 10.1039/c9ra02343g. eCollection 2019 Aug 13. RSC Adv. 2019. PMID: 35530078 Free PMC article.
-
Elucidating the molecular basis of spontaneous activation in an engineered mechanosensitive channel.Comput Struct Biotechnol J. 2022 May 23;20:2539-2550. doi: 10.1016/j.csbj.2022.05.022. eCollection 2022. Comput Struct Biotechnol J. 2022. PMID: 35685356 Free PMC article.
References
-
- Ajouz B, Berrier C, Garrigues A, Besnard M, Ghazi A. Release of thioredoxin via the mechanosensitive channel MscL during osmotic downshock of Escherichia coli cells. J Biol Chem. 1998;273:26670–26674. - PubMed
-
- Akitake B, Anishkin A, Liu N, Sukharev S. Straightening and sequential buckling of the pore-lining helices define the gating cycle of MscS. Nat Struct Mol Biol. 2007;14:1141–1149. - PubMed
-
- Andersen OS, Koeppe RE. Bilayer thickness and membrane protein function: An energetic perspective. Ann Rev Biophys Biomol Struct. 2007;36:107–130. - PubMed
Publication types
MeSH terms
Substances
Grants and funding
LinkOut - more resources
Full Text Sources
Molecular Biology Databases