Critical role for voltage-dependent anion channel 2 in infectious bursal disease virus-induced apoptosis in host cells via interaction with VP5
- PMID: 22114330
- PMCID: PMC3264341
- DOI: 10.1128/JVI.06104-11
Critical role for voltage-dependent anion channel 2 in infectious bursal disease virus-induced apoptosis in host cells via interaction with VP5
Abstract
Infectious bursal disease (IBD) is an acute, highly contagious, and immunosuppressive avian disease caused by IBD virus (IBDV). Although IBDV-induced host cell apoptosis has been established, the underlying molecular mechanism is still unclear. We report here that IBDV viral protein 5 (VP5) is a major apoptosis inducer in DF-1 cells by interacting with the voltage-dependent anion channel 2 (VDAC2) in the mitochondrion. We found that in DF-1 cells, VP5-induced apoptosis can be completely abolished by 4,4'-diisothiocyanatostibene-2,2'-disulfonic acid (DIDS), an inhibitor of VDAC. Furthermore, knockdown of VDAC2 by small interfering RNA markedly inhibits IBDV-induced apoptosis associated with decreased caspase-9 and -3 activation and cytochrome c release, leading to increased IBDV growth in host cells. Thus, VP5-induced apoptosis during IBDV infection is mediated by interacting with VDAC2, a protein that appears to restrict viral replication via induction of cell death.
Figures
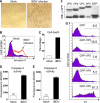
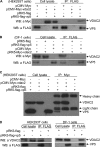
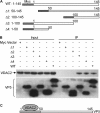
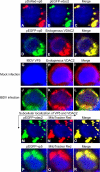
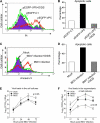
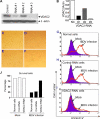
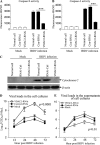
Similar articles
-
Role of MicroRNAs in Host Defense against Infectious Bursal Disease Virus (IBDV) Infection: A Hidden Front Line.Viruses. 2020 May 14;12(5):543. doi: 10.3390/v12050543. Viruses. 2020. PMID: 32423052 Free PMC article. Review.
-
Infectious Bursal Disease Virus-Host Interactions: Multifunctional Viral Proteins that Perform Multiple and Differing Jobs.Int J Mol Sci. 2017 Jan 14;18(1):161. doi: 10.3390/ijms18010161. Int J Mol Sci. 2017. PMID: 28098808 Free PMC article. Review.
-
The association of receptor of activated protein kinase C 1(RACK1) with infectious bursal disease virus viral protein VP5 and voltage-dependent anion channel 2 (VDAC2) inhibits apoptosis and enhances viral replication.J Biol Chem. 2015 Mar 27;290(13):8500-10. doi: 10.1074/jbc.M114.585687. Epub 2015 Jan 12. J Biol Chem. 2015. PMID: 25583988 Free PMC article.
-
Epigenetic Upregulation of Chicken MicroRNA-16-5p Expression in DF-1 Cells following Infection with Infectious Bursal Disease Virus (IBDV) Enhances IBDV-Induced Apoptosis and Viral Replication.J Virol. 2020 Jan 6;94(2):e01724-19. doi: 10.1128/JVI.01724-19. Print 2020 Jan 6. J Virol. 2020. PMID: 31694944 Free PMC article.
-
Voltage-Dependent Anion Channel 1 Interacts with Ribonucleoprotein Complexes To Enhance Infectious Bursal Disease Virus Polymerase Activity.J Virol. 2017 Jul 27;91(16):e00584-17. doi: 10.1128/JVI.00584-17. Print 2017 Aug 15. J Virol. 2017. PMID: 28592532 Free PMC article.
Cited by
-
VDAC2-specific cellular functions and the underlying structure.Biochim Biophys Acta. 2016 Oct;1863(10):2503-14. doi: 10.1016/j.bbamcr.2016.04.020. Epub 2016 Apr 23. Biochim Biophys Acta. 2016. PMID: 27116927 Free PMC article. Review.
-
Role of MicroRNAs in Host Defense against Infectious Bursal Disease Virus (IBDV) Infection: A Hidden Front Line.Viruses. 2020 May 14;12(5):543. doi: 10.3390/v12050543. Viruses. 2020. PMID: 32423052 Free PMC article. Review.
-
GHSC70 is involved in the cellular entry of nervous necrosis virus.J Virol. 2015 Jan;89(1):61-70. doi: 10.1128/JVI.02523-14. Epub 2014 Oct 15. J Virol. 2015. PMID: 25320288 Free PMC article.
-
Infectious Bursal Disease Virus-Host Interactions: Multifunctional Viral Proteins that Perform Multiple and Differing Jobs.Int J Mol Sci. 2017 Jan 14;18(1):161. doi: 10.3390/ijms18010161. Int J Mol Sci. 2017. PMID: 28098808 Free PMC article. Review.
-
The C-terminal amyloidogenic peptide contributes to self-assembly of Avibirnavirus viral protease.Sci Rep. 2015 Oct 6;5:14794. doi: 10.1038/srep14794. Sci Rep. 2015. PMID: 26440769 Free PMC article.
References
-
- Azad AA, Barrett SA, Fahey KJ. 1985. The characterization and molecular cloning of the double-stranded RNA genome of an Australian strain of infectious bursal disease virus. Virology 143: 35–44 - PubMed
-
- Cesar MC, Wilson JE. 2004. All three isoforms of the voltage-dependent anion channel (VDAC1, VDAC2, and VDAC3) are present in mitochondria from bovine, rabbit, and rat brain. Arch. Biochem. Biophys. 422: 191–196 - PubMed
-
- Cheng EH, Sheiko TV, Fisher JK, Craigen WJ, Korsmeyer SJ. 2003. VDAC2 inhibits BAK activation and mitochondrial apoptosis. Science 301: 513–517 - PubMed
Publication types
MeSH terms
Substances
LinkOut - more resources
Full Text Sources
Miscellaneous