Differentiation of the DnaA-oriC subcomplex for DNA unwinding in a replication initiation complex
- PMID: 22942281
- PMCID: PMC3481341
- DOI: 10.1074/jbc.M112.372052
Differentiation of the DnaA-oriC subcomplex for DNA unwinding in a replication initiation complex
Abstract
In Escherichia coli, ATP-DnaA multimers formed on the replication origin oriC promote duplex unwinding, which leads to helicase loading. Based on a detailed functional analysis of the oriC sequence motifs, we previously proposed that the left half of oriC forms an ATP-DnaA subcomplex competent for oriC unwinding, whereas the right half of oriC forms a distinct ATP-DnaA subcomplex that facilitates helicase loading. However, the molecular basis for the functional difference between these ATP-DnaA subcomplexes remains unclear. By analyzing a series of novel DnaA mutants, we found that structurally distinct DnaA multimers form on each half of oriC. DnaA AAA+ domain residues Arg-227 and Leu-290 are specifically required for oriC unwinding. Notably, these residues are required for the ATP-DnaA-specific structure of DnaA multimers in complex with the left half of oriC but not for that with the right half. These results support the idea that the ATP-DnaA multimers formed on oriC are not uniform and that they can adopt different conformations. Based on a structural model, we propose that Arg-227 and Leu-290 play a crucial role in inter-ATP-DnaA interaction and are a prerequisite for the formation of unwinding-competent DnaA subcomplexes on the left half of oriC. These residues are not required for the interaction with DnaB, nucleotide binding, or regulatory DnaA-ATP hydrolysis, which further supports their important role in inter-DnaA interaction. The corresponding residues are evolutionarily conserved and are required for unwinding in the initial complexes of Thermotoga maritima, an ancient hyperthermophile. Therefore, our findings suggest a novel and common mechanism for ATP-DnaA-dependent activation of initial complexes.
Figures
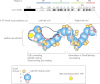
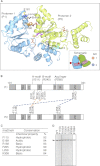
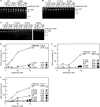
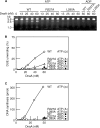
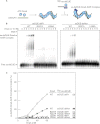
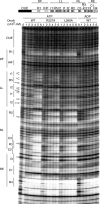
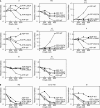
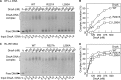
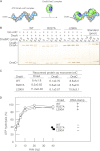
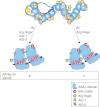
Similar articles
-
A common mechanism for the ATP-DnaA-dependent formation of open complexes at the replication origin.J Biol Chem. 2008 Mar 28;283(13):8351-62. doi: 10.1074/jbc.M708684200. Epub 2008 Jan 23. J Biol Chem. 2008. PMID: 18216012
-
Concerted actions of DnaA complexes with DNA-unwinding sequences within and flanking replication origin oriC promote DnaB helicase loading.J Biol Chem. 2022 Jun;298(6):102051. doi: 10.1016/j.jbc.2022.102051. Epub 2022 May 19. J Biol Chem. 2022. PMID: 35598828 Free PMC article.
-
Near-atomic structural model for bacterial DNA replication initiation complex and its functional insights.Proc Natl Acad Sci U S A. 2016 Dec 13;113(50):E8021-E8030. doi: 10.1073/pnas.1609649113. Epub 2016 Nov 29. Proc Natl Acad Sci U S A. 2016. PMID: 27911788 Free PMC article.
-
DnaA structure, function, and dynamics in the initiation at the chromosomal origin.Plasmid. 2009 Sep;62(2):71-82. doi: 10.1016/j.plasmid.2009.06.003. Epub 2009 Jun 13. Plasmid. 2009. PMID: 19527752 Review.
-
Initiation of DNA Replication at the Chromosomal Origin of E. coli, oriC.Adv Exp Med Biol. 2017;1042:79-98. doi: 10.1007/978-981-10-6955-0_4. Adv Exp Med Biol. 2017. PMID: 29357054 Review.
Cited by
-
Lon recognition of the replication initiator DnaA requires a bipartite degron.Mol Microbiol. 2019 Jan;111(1):176-186. doi: 10.1111/mmi.14146. Epub 2018 Nov 8. Mol Microbiol. 2019. PMID: 30288816 Free PMC article.
-
The Role of the N-Terminal Domains of Bacterial Initiator DnaA in the Assembly and Regulation of the Bacterial Replication Initiation Complex.Genes (Basel). 2017 May 10;8(5):136. doi: 10.3390/genes8050136. Genes (Basel). 2017. PMID: 28489024 Free PMC article. Review.
-
Interactions of replication initiator RctB with single- and double-stranded DNA in origin opening of Vibrio cholerae chromosome 2.Nucleic Acids Res. 2020 Nov 4;48(19):11016-11029. doi: 10.1093/nar/gkaa826. Nucleic Acids Res. 2020. PMID: 33035310 Free PMC article.
-
Timely binding of IHF and Fis to DARS2 regulates ATP-DnaA production and replication initiation.Nucleic Acids Res. 2014 Dec 1;42(21):13134-49. doi: 10.1093/nar/gku1051. Epub 2014 Nov 6. Nucleic Acids Res. 2014. PMID: 25378325 Free PMC article.
-
Identification of the Unwinding Region in the Clostridioides difficile Chromosomal Origin of Replication.Front Microbiol. 2020 Oct 2;11:581401. doi: 10.3389/fmicb.2020.581401. eCollection 2020. Front Microbiol. 2020. PMID: 33133049 Free PMC article.
References
-
- Bramhill D., Kornberg A. (1988) Duplex opening by dnaA protein at novel sequences in initiation of replication at the origin of the E. coli chromosome. Cell 52, 743–755 - PubMed
-
- Messer W. (2002) The bacterial replication initiator DnaA. DnaA and oriC, the bacterial mode to initiate DNA replication. FEMS Microbiol. Rev. 26, 355–374 - PubMed
-
- Duderstadt K. E., Berger J. M. (2008) AAA+ ATPases in the initiation of DNA replication. Crit. Rev. Biochem. Mol. Biol. 43, 163–187 - PubMed
-
- Ozaki S., Katayama T. (2009) DnaA structure, function, and dynamics in the initiation at the chromosomal origin. Plasmid 62, 71–82 - PubMed
Publication types
MeSH terms
Substances
LinkOut - more resources
Full Text Sources
Molecular Biology Databases