Structure-guided simulations illuminate the mechanism of ATP transport through VDAC1
- PMID: 24908397
- PMCID: PMC4157756
- DOI: 10.1038/nsmb.2841
Structure-guided simulations illuminate the mechanism of ATP transport through VDAC1
Abstract
The voltage-dependent anion channel (VDAC) mediates the flow of metabolites and ions across the outer mitochondrial membrane of all eukaryotic cells. The open channel passes millions of ATP molecules per second, whereas the closed state exhibits no detectable ATP flux. High-resolution structures of VDAC1 revealed a 19-stranded β-barrel with an α-helix partially occupying the central pore. To understand ATP permeation through VDAC, we solved the crystal structure of mouse VDAC1 (mVDAC1) in the presence of ATP, revealing a low-affinity binding site. Guided by these coordinates, we initiated hundreds of molecular dynamics simulations to construct a Markov state model of ATP permeation. These simulations indicate that ATP flows through VDAC through multiple pathways, in agreement with our structural data and experimentally determined physiological rates.
Conflict of interest statement
The authors declare no competing interests.
Figures
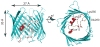
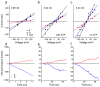
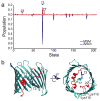
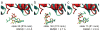
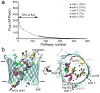
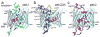
Comment in
-
Channeling your inner energy.Nat Struct Mol Biol. 2014 Jul;21(7):575-7. doi: 10.1038/nsmb.2854. Nat Struct Mol Biol. 2014. PMID: 24992223 No abstract available.
Similar articles
-
ATP transport through VDAC and the VDAC-tubulin complex probed by equilibrium and nonequilibrium MD simulations.Biochemistry. 2013 Dec 23;52(51):9246-56. doi: 10.1021/bi4011495. Epub 2013 Nov 25. Biochemistry. 2013. PMID: 24245503 Free PMC article.
-
An N-terminal nucleotide-binding site in VDAC1: involvement in regulating mitochondrial function.J Cell Physiol. 2007 Aug;212(2):551-61. doi: 10.1002/jcp.21048. J Cell Physiol. 2007. PMID: 17503466
-
The crystal structure of mouse VDAC1 at 2.3 A resolution reveals mechanistic insights into metabolite gating.Proc Natl Acad Sci U S A. 2008 Nov 18;105(46):17742-7. doi: 10.1073/pnas.0809634105. Epub 2008 Nov 6. Proc Natl Acad Sci U S A. 2008. PMID: 18988731 Free PMC article.
-
The mitochondrial voltage-dependent anion channel 1 in tumor cells.Biochim Biophys Acta. 2015 Oct;1848(10 Pt B):2547-75. doi: 10.1016/j.bbamem.2014.10.040. Epub 2014 Nov 4. Biochim Biophys Acta. 2015. PMID: 25448878 Review.
-
Role of cysteines in mammalian VDAC isoforms' function.Biochim Biophys Acta. 2016 Aug;1857(8):1219-1227. doi: 10.1016/j.bbabio.2016.02.020. Epub 2016 Mar 4. Biochim Biophys Acta. 2016. PMID: 26947058 Free PMC article. Review.
Cited by
-
Structure, gating and interactions of the voltage-dependent anion channel.Eur Biophys J. 2021 Mar;50(2):159-172. doi: 10.1007/s00249-021-01515-7. Epub 2021 Mar 29. Eur Biophys J. 2021. PMID: 33782728 Free PMC article. Review.
-
Post-translational modifications and protein quality control of mitochondrial channels and transporters.Front Cell Dev Biol. 2023 Aug 3;11:1196466. doi: 10.3389/fcell.2023.1196466. eCollection 2023. Front Cell Dev Biol. 2023. PMID: 37601094 Free PMC article. Review.
-
Protein Ensembles: How Does Nature Harness Thermodynamic Fluctuations for Life? The Diverse Functional Roles of Conformational Ensembles in the Cell.Chem Rev. 2016 Jun 8;116(11):6516-51. doi: 10.1021/acs.chemrev.5b00562. Epub 2016 Jan 25. Chem Rev. 2016. PMID: 26807783 Free PMC article. Review.
-
News about VDAC1 in Hypoxia.Front Oncol. 2016 Aug 30;6:193. doi: 10.3389/fonc.2016.00193. eCollection 2016. Front Oncol. 2016. PMID: 27625993 Free PMC article.
-
Studying membrane proteins with MicroED.Biochem Soc Trans. 2022 Feb 28;50(1):231-239. doi: 10.1042/BST20210911. Biochem Soc Trans. 2022. PMID: 35191473 Free PMC article.
References
-
- Schwarzer C, Barnikol-Watanabe S, Thinnes FP, Hilschmann N. Voltage-dependent anion-selective channel (VDAC) interacts with the dynein light chain Tctex1 and the heat-shock protein PBP74. Int J Biochem Cell Biol. 2002;34:1059–70. - PubMed
-
- Shimizu S, Narita M, Tsujimoto Y. Bcl-2 family proteins regulate the release of apoptogenic cytochrome c by the mitochondrial channel VDAC. Nature. 1999;399:483–7. - PubMed
-
- Min CK, et al. Coupling of ryanodine receptor 2 and voltage-dependent anion channel 2 is essential for Ca(2)+ transfer from the sarcoplasmic reticulum to the mitochondria in the heart. Biochem J. 2012;447:371–9. - PubMed
Publication types
MeSH terms
Substances
Associated data
- Actions
Grants and funding
LinkOut - more resources
Full Text Sources
Other Literature Sources