Crystal structure of the eukaryotic origin recognition complex
- PMID: 25762138
- PMCID: PMC4368505
- DOI: 10.1038/nature14239
Crystal structure of the eukaryotic origin recognition complex
Abstract
Initiation of cellular DNA replication is tightly controlled to sustain genomic integrity. In eukaryotes, the heterohexameric origin recognition complex (ORC) is essential for coordinating replication onset. Here we describe the crystal structure of Drosophila ORC at 3.5 Å resolution, showing that the 270 kilodalton initiator core complex comprises a two-layered notched ring in which a collar of winged-helix domains from the Orc1-5 subunits sits atop a layer of AAA+ (ATPases associated with a variety of cellular activities) folds. Although canonical inter-AAA+ domain interactions exist between four of the six ORC subunits, unanticipated features are also evident. These include highly interdigitated domain-swapping interactions between the winged-helix folds and AAA+ modules of neighbouring protomers, and a quasi-spiral arrangement of DNA binding elements that circumnavigate an approximately 20 Å wide channel in the centre of the complex. Comparative analyses indicate that ORC encircles DNA, using its winged-helix domain face to engage the mini-chromosome maintenance 2-7 (MCM2-7) complex during replicative helicase loading; however, an observed out-of-plane rotation of more than 90° for the Orc1 AAA+ domain disrupts interactions with catalytic amino acids in Orc4, narrowing and sealing off entry into the central channel. Prima facie, our data indicate that Drosophila ORC can switch between active and autoinhibited conformations, suggesting a novel means for cell cycle and/or developmental control of ORC functions.
Figures
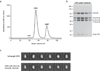
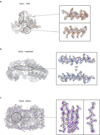
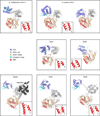
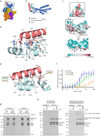
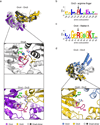
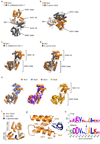
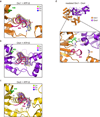
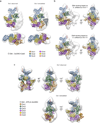
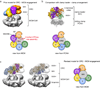
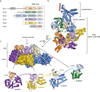
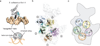
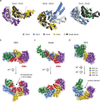
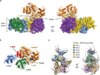
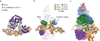
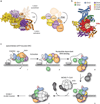
Similar articles
-
Conformational control and DNA-binding mechanism of the metazoan origin recognition complex.Proc Natl Acad Sci U S A. 2018 Jun 26;115(26):E5906-E5915. doi: 10.1073/pnas.1806315115. Epub 2018 Jun 13. Proc Natl Acad Sci U S A. 2018. PMID: 29899147 Free PMC article.
-
Structural mechanism for replication origin binding and remodeling by a metazoan origin recognition complex and its co-loader Cdc6.Nat Commun. 2020 Aug 26;11(1):4263. doi: 10.1038/s41467-020-18067-7. Nat Commun. 2020. PMID: 32848132 Free PMC article.
-
The regulatory function of N-terminal AAA+ ATPase domain of eukaryote-like archaeal Orc1/Cdc6 protein during DNA replication initiation.Arch Biochem Biophys. 2008 Mar 15;471(2):176-83. doi: 10.1016/j.abb.2008.01.007. Epub 2008 Jan 19. Arch Biochem Biophys. 2008. PMID: 18237540
-
Unique Roles of the Non-identical MCM Subunits in DNA Replication Licensing.Mol Cell. 2017 Jul 20;67(2):168-179. doi: 10.1016/j.molcel.2017.06.016. Mol Cell. 2017. PMID: 28732205 Review.
-
The origin recognition complex protein family.Genome Biol. 2009;10(3):214. doi: 10.1186/gb-2009-10-3-214. Epub 2009 Mar 17. Genome Biol. 2009. PMID: 19344485 Free PMC article. Review.
Cited by
-
Evolution of DNA replication origin specification and gene silencing mechanisms.Nat Commun. 2020 Oct 14;11(1):5175. doi: 10.1038/s41467-020-18964-x. Nat Commun. 2020. PMID: 33056978 Free PMC article.
-
Structure of the eukaryotic MCM complex at 3.8 Å.Nature. 2015 Aug 13;524(7564):186-91. doi: 10.1038/nature14685. Epub 2015 Jul 29. Nature. 2015. PMID: 26222030
-
Drosophila model of Meier-Gorlin syndrome based on the mutation in a conserved C-Terminal domain of Orc6.Am J Med Genet A. 2015 Nov;167A(11):2533-40. doi: 10.1002/ajmg.a.37214. Epub 2015 Jul 2. Am J Med Genet A. 2015. PMID: 26139588 Free PMC article.
-
Cryo-EM of dynamic protein complexes in eukaryotic DNA replication.Protein Sci. 2017 Jan;26(1):40-51. doi: 10.1002/pro.3033. Epub 2016 Sep 14. Protein Sci. 2017. PMID: 27589669 Free PMC article. Review.
-
Novel candidate biomarkers of origin recognition complex 1, 5 and 6 for survival surveillance in patients with hepatocellular carcinoma.J Cancer. 2020 Jan 20;11(7):1869-1882. doi: 10.7150/jca.39163. eCollection 2020. J Cancer. 2020. PMID: 32194798 Free PMC article.
References
-
- Neuwald AF, Aravind L, Spouge JL, Koonin EV. AAA+: A class of chaperone-like ATPases associated with the assembly, operation, and disassembly of protein complexes. Genome Res. 1999;9:27–43. - PubMed
-
- Bell SP, Stillman B. ATP-dependent recognition of eukaryotic origins of DNA replication by a multiprotein complex. Nature. 1992;357:128–134. - PubMed
Publication types
MeSH terms
Substances
Associated data
- Actions
Grants and funding
LinkOut - more resources
Full Text Sources
Other Literature Sources
Molecular Biology Databases
Miscellaneous