Maintenance of electrostatic stabilization in altered tubulin lateral contacts may facilitate formation of helical filaments in foraminifera
- PMID: 27539392
- PMCID: PMC4990898
- DOI: 10.1038/srep31723
Maintenance of electrostatic stabilization in altered tubulin lateral contacts may facilitate formation of helical filaments in foraminifera
Abstract
Microtubules in foraminiferan protists (forams) can convert into helical filament structures, in which longitudinal intraprotofilament interactions between tubulin heterodimers are thought to be lost, while lateral contacts across protofilaments are still maintained. The coarse geometric features of helical filaments are known through low-resolution negative stain electron microscopy (EM). In this study, geometric restraints derived from these experimental data were used to generate an average atomic-scale helical filament model, which anticipated a modest reorientation in the lateral tubulin heterodimer interface. Restrained molecular dynamics (MD) simulations of the nearest neighbor interactions combined with a Genalized Born implicit solvent model were used to assess the lateral, longitudinal, and seam contacts in 13-3 microtubules and the reoriented lateral contacts in the helical filament model. This electrostatic analysis suggests that the change in the lateral interface in the helical filament does not greatly diminish the lateral electrostatic interaction. After longitudinal dissociation, the 13-3 seam interaction is much weaker than the reoriented lateral interface in the helical filament model, providing a plausible atomic-detail explanation for seam-to-lateral contact transition that enables the transition to a helical filament structure.
Figures
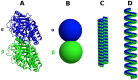
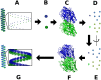
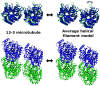
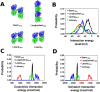
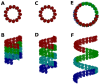
Similar articles
-
Molecular evidence for β-tubulin neofunctionalization in Retaria (Foraminifera and radiolarians).Mol Biol Evol. 2013 Nov;30(11):2487-93. doi: 10.1093/molbev/mst150. Epub 2013 Sep 4. Mol Biol Evol. 2013. PMID: 24008583
-
Microtubules dual chemo and thermo-responsive depolymerization.Proteins. 2015 May;83(5):970-81. doi: 10.1002/prot.24793. Epub 2015 Mar 25. Proteins. 2015. PMID: 25739855
-
Evidence for a direct conversion between two tubulin polymers--microtubules and helical filaments--in the foraminiferan, Allogromia laticollaris.Cell Motil Cytoskeleton. 1998;41(2):107-16. doi: 10.1002/(SICI)1097-0169(1998)41:23.0.CO;2-B. Cell Motil Cytoskeleton. 1998. PMID: 9786086
-
Tubulin lattice in cilia is in a stressed form regulated by microtubule inner proteins.Proc Natl Acad Sci U S A. 2019 Oct 1;116(40):19930-19938. doi: 10.1073/pnas.1911119116. Epub 2019 Sep 16. Proc Natl Acad Sci U S A. 2019. PMID: 31527277 Free PMC article.
-
An electron microscopy journey in the study of microtubule structure and dynamics.Protein Sci. 2015 Dec;24(12):1912-9. doi: 10.1002/pro.2808. Epub 2015 Oct 11. Protein Sci. 2015. PMID: 26401895 Free PMC article. Review.
Cited by
-
Myosin B of Plasmodium falciparum (PfMyoB): in silico prediction of its three-dimensional structure and its possible interaction with MTIP.Parasitol Res. 2017 Apr;116(4):1373-1382. doi: 10.1007/s00436-017-5417-y. Epub 2017 Mar 7. Parasitol Res. 2017. PMID: 28265752
-
Adsorption of a Helical Filament Subject to Thermal Fluctuations.Polymers (Basel). 2020 Jan 10;12(1):192. doi: 10.3390/polym12010192. Polymers (Basel). 2020. PMID: 31936860 Free PMC article.
References
-
- Avila J. Microtubule functions. Life Sciences 50, 327–334 (1992). - PubMed
-
- Tsutsui H. et al.. Role of microtubules in contractile dysfunction of hypertrophied cardiocytes. Circulation 90, 533–555 (1994). - PubMed
-
- Zhou J. & Giannakakou P. Targeting microtubules for cancer chemotherapy. Current Medicinal Chemistry - Anti-Cancer Agents 5, 65–71 (2005). - PubMed
-
- Nogales E., Wolf S. & Downing K. Structure of the αβ-tubulin dimer by electron crystallography. Nature 199–202 (1998). - PubMed
Publication types
MeSH terms
Substances
LinkOut - more resources
Full Text Sources
Other Literature Sources