Diverse feather shape evolution enabled by coupling anisotropic signalling modules with self-organizing branching programme
- PMID: 28106042
- PMCID: PMC5263876
- DOI: 10.1038/ncomms14139
Diverse feather shape evolution enabled by coupling anisotropic signalling modules with self-organizing branching programme
Abstract
Adaptation of feathered dinosaurs and Mesozoic birds to new ecological niches was potentiated by rapid diversification of feather vane shapes. The molecular mechanism driving this spectacular process remains unclear. Here, through morphology analysis, transcriptome profiling, functional perturbations and mathematical simulations, we find that mesenchyme-derived GDF10 and GREM1 are major controllers for the topologies of rachidial and barb generative zones (setting vane boundaries), respectively, by tuning the periodic-branching programme of epithelial progenitors. Their interactions with the anterior-posterior WNT gradient establish the bilateral-symmetric vane configuration. Additionally, combinatory effects of CYP26B1, CRABP1 and RALDH3 establish dynamic retinoic acid (RA) landscapes in feather mesenchyme, which modulate GREM1 expression and epithelial cell shapes. Incremental changes of RA gradient slopes establish a continuum of asymmetric flight feathers along the wing, while switch-like modulation of RA signalling confers distinct vane shapes between feather tracts. Therefore, the co-option of anisotropic signalling modules introduced new dimensions of feather shape diversification.
Figures
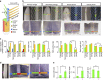
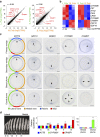
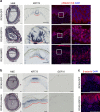
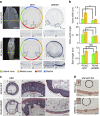
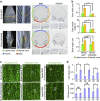
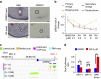
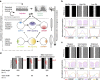
Similar articles
-
Barb geometry of asymmetrical feathers reveals a transitional morphology in the evolution of avian flight.Proc Biol Sci. 2015 Mar 22;282(1803):20142864. doi: 10.1098/rspb.2014.2864. Proc Biol Sci. 2015. PMID: 25673687 Free PMC article.
-
Theoretical morphology and development of flight feather vane asymmetry with experimental tests in parrots.J Exp Zool B Mol Dev Evol. 2014 Jun;322(4):240-55. doi: 10.1002/jez.b.22573. Epub 2014 May 9. J Exp Zool B Mol Dev Evol. 2014. PMID: 24816758
-
The Making of a Flight Feather: Bio-architectural Principles and Adaptation.Cell. 2019 Nov 27;179(6):1409-1423.e17. doi: 10.1016/j.cell.2019.11.008. Cell. 2019. PMID: 31778655 Free PMC article.
-
Adaptation to the sky: Defining the feather with integument fossils from mesozoic China and experimental evidence from molecular laboratories.J Exp Zool B Mol Dev Evol. 2003 Aug 15;298(1):42-56. doi: 10.1002/jez.b.25. J Exp Zool B Mol Dev Evol. 2003. PMID: 12949768 Free PMC article. Review.
-
The evolutionary origin and diversification of feathers.Q Rev Biol. 2002 Sep;77(3):261-95. doi: 10.1086/341993. Q Rev Biol. 2002. PMID: 12365352 Review.
Cited by
-
Cassowary gloss and a novel form of structural color in birds.Sci Adv. 2020 May 13;6(20):eaba0187. doi: 10.1126/sciadv.aba0187. eCollection 2020 May. Sci Adv. 2020. PMID: 32426504 Free PMC article.
-
The Wnt/β-catenin signaling pathway is involved in regulating feather growth of embryonic chicks.Poult Sci. 2020 May;99(5):2315-2323. doi: 10.1016/j.psj.2020.01.002. Epub 2020 Mar 18. Poult Sci. 2020. PMID: 32359566 Free PMC article.
-
Combined transcriptomics and proteomics forecast analysis for potential genes regulating the Columbian plumage color in chickens.PLoS One. 2019 Nov 6;14(11):e0210850. doi: 10.1371/journal.pone.0210850. eCollection 2019. PLoS One. 2019. PMID: 31693656 Free PMC article.
-
Hypothesis: Wound-induced TLR3 activation stimulates endogenous retinoic acid synthesis and signalling during regeneration.Exp Dermatol. 2019 Apr;28(4):450-452. doi: 10.1111/exd.13931. Exp Dermatol. 2019. PMID: 30927295 Free PMC article.
-
Molecular Signaling and Nutritional Regulation in the Context of Poultry Feather Growth and Regeneration.Front Physiol. 2020 Jan 21;10:1609. doi: 10.3389/fphys.2019.01609. eCollection 2019. Front Physiol. 2020. PMID: 32038289 Free PMC article. Review.
References
-
- Prum R. O. & Brush A. H. The evolutionary origin and diversification of feathers. Q. Rev. Biol. 77, 261–295 (2002). - PubMed
-
- Xu X. et al.. An integrative approach to understanding bird origins. Science 346, 1253293 (2014). - PubMed
-
- Xu X., Zheng X. & You H. Exceptional dinosaur fossils show ontogenetic development of early feathers. Nature 464, 1338–1341 (2010). - PubMed
-
- Chiappe L. M. in Glorified Dinosaurs: The Origin and Early Evolution of Birds 263, John Wiley (2007).
Publication types
MeSH terms
Substances
Grants and funding
LinkOut - more resources
Full Text Sources
Other Literature Sources
Molecular Biology Databases
Research Materials