Regulatory dynamics in the ternary DnaA complex for initiation of chromosomal replication in Escherichia coli
- PMID: 29040689
- PMCID: PMC5716108
- DOI: 10.1093/nar/gkx914
Regulatory dynamics in the ternary DnaA complex for initiation of chromosomal replication in Escherichia coli
Abstract
In Escherichia coli, the level of the ATP-DnaA initiator is increased temporarily at the time of replication initiation. The replication origin, oriC, contains a duplex-unwinding element (DUE) flanking a DnaA-oligomerization region (DOR), which includes twelve DnaA-binding sites (DnaA boxes) and the DNA-bending protein IHF-binding site (IBS). Although complexes of IHF and ATP-DnaA assembly on the DOR unwind the DUE, the configuration of the crucial nucleoprotein complexes remains elusive. To resolve this, we analyzed individual DnaA protomers in the complex and here demonstrate that the DUE-DnaA-box-R1-IBS-DnaA-box-R5M region is essential for DUE unwinding. R5M-bound ATP-DnaA predominantly promotes ATP-DnaA assembly on the DUE-proximal DOR, and R1-bound DnaA has a supporting role. This mechanism might support timely assembly of ATP-DnaA on oriC. DnaA protomers bound to R1 and R5M directly bind to the unwound DUE strand, which is crucial in replication initiation. Data from in vivo experiments support these results. We propose that the DnaA assembly on the IHF-bent DOR directly binds to the unwound DUE strand, and timely formation of this ternary complex regulates replication initiation. Structural features of oriC support the idea that these mechanisms for DUE unwinding are fundamentally conserved in various bacterial species including pathogens.
© The Author(s) 2017. Published by Oxford University Press on behalf of Nucleic Acids Research.
Figures
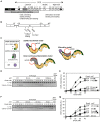
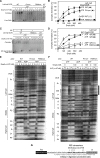
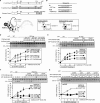
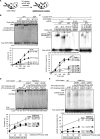
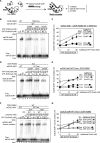
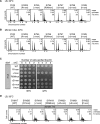
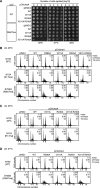
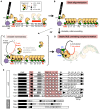
Similar articles
-
Single-stranded DNA recruitment mechanism in replication origin unwinding by DnaA initiator protein and HU, an evolutionary ubiquitous nucleoid protein.Nucleic Acids Res. 2023 Jul 7;51(12):6286-6306. doi: 10.1093/nar/gkad389. Nucleic Acids Res. 2023. PMID: 37178000 Free PMC article.
-
Escherichia coli prereplication complex assembly is regulated by dynamic interplay among Fis, IHF and DnaA.Mol Microbiol. 2004 Mar;51(5):1347-59. doi: 10.1046/j.1365-2958.2003.03906.x. Mol Microbiol. 2004. PMID: 14982629
-
IHF and Fis as Escherichia coli Cell Cycle Regulators: Activation of the Replication Origin oriC and the Regulatory Cycle of the DnaA Initiator.Int J Mol Sci. 2023 Jul 18;24(14):11572. doi: 10.3390/ijms241411572. Int J Mol Sci. 2023. PMID: 37511331 Free PMC article. Review.
-
Timely binding of IHF and Fis to DARS2 regulates ATP-DnaA production and replication initiation.Nucleic Acids Res. 2014 Dec 1;42(21):13134-49. doi: 10.1093/nar/gku1051. Epub 2014 Nov 6. Nucleic Acids Res. 2014. PMID: 25378325 Free PMC article.
-
The DnaA Cycle in Escherichia coli: Activation, Function and Inactivation of the Initiator Protein.Front Microbiol. 2017 Dec 21;8:2496. doi: 10.3389/fmicb.2017.02496. eCollection 2017. Front Microbiol. 2017. PMID: 29312202 Free PMC article. Review.
Cited by
-
Low Affinity DnaA-ATP Recognition Sites in E. coli oriC Make Non-equivalent and Growth Rate-Dependent Contributions to the Regulated Timing of Chromosome Replication.Front Microbiol. 2018 Jul 26;9:1673. doi: 10.3389/fmicb.2018.01673. eCollection 2018. Front Microbiol. 2018. PMID: 30093890 Free PMC article.
-
Rep protein accommodates together dsDNA and ssDNA which enables a loop-back mechanism to plasmid DNA replication initiation.Nucleic Acids Res. 2023 Oct 27;51(19):10551-10567. doi: 10.1093/nar/gkad740. Nucleic Acids Res. 2023. PMID: 37713613 Free PMC article.
-
Open Questions about the Roles of DnaA, Related Proteins, and Hyperstructure Dynamics in the Cell Cycle.Life (Basel). 2023 Sep 10;13(9):1890. doi: 10.3390/life13091890. Life (Basel). 2023. PMID: 37763294 Free PMC article. Review.
-
Changing Perspectives on the Role of DnaA-ATP in Orisome Function and Timing Regulation.Front Microbiol. 2019 Aug 29;10:2009. doi: 10.3389/fmicb.2019.02009. eCollection 2019. Front Microbiol. 2019. PMID: 31555240 Free PMC article. Review.
-
The bacterial cell cycle, chromosome inheritance and cell growth.Nat Rev Microbiol. 2019 Aug;17(8):467-478. doi: 10.1038/s41579-019-0212-7. Nat Rev Microbiol. 2019. PMID: 31164753 Review.
References
-
- Katayama T., Ozaki S., Keyamura K., Fujimitsu K.. Regulation of the replication cycle: conserved and diverse regulatory systems for DnaA and oriC. Nat. Rev. Microbiol. 2010; 8:163–170. - PubMed
MeSH terms
Substances
LinkOut - more resources
Full Text Sources
Other Literature Sources
Molecular Biology Databases