Neurodegenerative Charcot-Marie-Tooth disease as a case study to decipher novel functions of aminoacyl-tRNA synthetases
- PMID: 30643024
- PMCID: PMC6462521
- DOI: 10.1074/jbc.REV118.002955
Neurodegenerative Charcot-Marie-Tooth disease as a case study to decipher novel functions of aminoacyl-tRNA synthetases
Abstract
Aminoacyl-tRNA synthetases (aaRSs) are essential enzymes that catalyze the first reaction in protein biosynthesis, namely the charging of transfer RNAs (tRNAs) with their cognate amino acids. aaRSs have been increasingly implicated in dominantly and recessively inherited human diseases. The most common aaRS-associated monogenic disorder is the incurable neurodegenerative disease Charcot-Marie-Tooth neuropathy (CMT), caused by dominant mono-allelic mutations in aaRSs. With six currently known members (GlyRS, TyrRS, AlaRS, HisRS, TrpRS, and MetRS), aaRSs represent the largest protein family implicated in CMT etiology. After the initial discovery linking aaRSs to CMT, the field has progressed from understanding whether impaired tRNA charging is a critical component of this disease to elucidating the specific pathways affected by CMT-causing mutations in aaRSs. Although many aaRS CMT mutants result in loss of tRNA aminoacylation function, animal genetics studies demonstrated that dominant mutations in GlyRS cause CMT through toxic gain-of-function effects, which also may apply to other aaRS-linked CMT subtypes. The CMT-causing mechanism is likely to be multifactorial and involves multiple cellular compartments, including the nucleus and the extracellular space, where the normal WT enzymes also appear. Thus, the association of aaRSs with neuropathy is relevant to discoveries indicating that aaRSs also have nonenzymatic regulatory functions that coordinate protein synthesis with other biological processes. Through genetic, functional, and structural analyses, commonalities among different mutations and different aaRS-linked CMT subtypes have begun to emerge, providing insights into the nonenzymatic functions of aaRSs and the pathogenesis of aaRS-linked CMT to guide therapeutic development to treat this disease.
Keywords: aminoacyl tRNA synthetase; animal model; drug development; genetic disease; neurodegenerative disease; pathogenesis; protein structure; protein synthesis; transfer RNA (tRNA); translation.
© 2019 Wei et al.
Conflict of interest statement
The authors declare that they have no conflicts of interest with the contents of this article
Figures
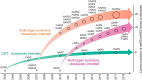
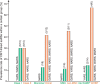
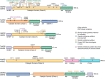
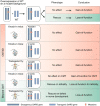
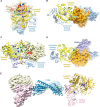
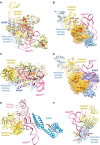
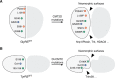
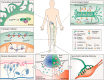
Similar articles
-
CMT disease severity correlates with mutation-induced open conformation of histidyl-tRNA synthetase, not aminoacylation loss, in patient cells.Proc Natl Acad Sci U S A. 2019 Sep 24;116(39):19440-19448. doi: 10.1073/pnas.1908288116. Epub 2019 Sep 9. Proc Natl Acad Sci U S A. 2019. PMID: 31501329 Free PMC article.
-
Aminoacyl-tRNA synthetases in Charcot-Marie-Tooth disease: A gain or a loss?J Neurochem. 2021 May;157(3):351-369. doi: 10.1111/jnc.15249. Epub 2020 Dec 19. J Neurochem. 2021. PMID: 33236345 Free PMC article. Review.
-
Associations between Neurological Diseases and Mutations in the Human Glycyl-tRNA Synthetase.Biochemistry (Mosc). 2021 Jan;86(Suppl 1):S12-S23. doi: 10.1134/S0006297921140029. Biochemistry (Mosc). 2021. PMID: 33827397 Free PMC article. Review.
-
Human diseases linked to cytoplasmic aminoacyl-tRNA synthetases.Enzymes. 2020;48:277-319. doi: 10.1016/bs.enz.2020.06.009. Epub 2020 Sep 28. Enzymes. 2020. PMID: 33837707
-
Peripheral neuropathy via mutant tRNA synthetases: Inhibition of protein translation provides a possible explanation.Bioessays. 2016 Sep;38(9):818-29. doi: 10.1002/bies.201600052. Epub 2016 Jun 28. Bioessays. 2016. PMID: 27352040 Free PMC article. Review.
Cited by
-
HDAC6 Inhibition Corrects Electrophysiological and Axonal Transport Deficits in a Human Stem Cell-Based Model of Charcot-Marie-Tooth Disease (Type 2D).Adv Biol (Weinh). 2022 Feb;6(2):e2101308. doi: 10.1002/adbi.202101308. Epub 2021 Dec 26. Adv Biol (Weinh). 2022. PMID: 34958183 Free PMC article.
-
Translation dysregulation in neurodegenerative diseases: a focus on ALS.Mol Neurodegener. 2023 Aug 25;18(1):58. doi: 10.1186/s13024-023-00642-3. Mol Neurodegener. 2023. PMID: 37626421 Free PMC article. Review.
-
An asymmetric structure of bacterial TrpRS supports the half-of-the-sites catalytic mechanism and facilitates antimicrobial screening.Nucleic Acids Res. 2023 May 22;51(9):4637-4649. doi: 10.1093/nar/gkad278. Nucleic Acids Res. 2023. PMID: 37070195 Free PMC article.
-
Tyrosyl-tRNA synthetase has a noncanonical function in actin bundling.Nat Commun. 2023 Mar 8;14(1):999. doi: 10.1038/s41467-023-35908-3. Nat Commun. 2023. PMID: 36890170 Free PMC article.
-
Alanyl-tRNA Synthetase 1 Gene Variants in Hereditary Neuropathy: Genotype and Phenotype Overview.Neurol Genet. 2022 Sep 5;8(5):e200019. doi: 10.1212/NXG.0000000000200019. eCollection 2022 Oct. Neurol Genet. 2022. PMID: 36092982 Free PMC article.
References
Publication types
MeSH terms
Substances
Associated data
- Actions
- Actions
- Actions
- Actions
- Actions
- Actions
- Actions
- Actions
- Actions
- Actions
- Actions
- Actions
- Actions
- Actions
Grants and funding
LinkOut - more resources
Full Text Sources
Other Literature Sources
Medical
Miscellaneous