Trypanosoma cruzi surface mucins are involved in the attachment to the Triatoma infestans rectal ampoule
- PMID: 31107901
- PMCID: PMC6544316
- DOI: 10.1371/journal.pntd.0007418
Trypanosoma cruzi surface mucins are involved in the attachment to the Triatoma infestans rectal ampoule
Abstract
Background: Trypanosoma cruzi, the agent of Chagas disease, is a protozoan parasite transmitted to humans by blood-sucking triatomine vectors. However, and despite its utmost biological and epidemiological relevance, T. cruzi development inside the digestive tract of the insect remains a poorly understood process.
Methods/principle findings: Here we showed that Gp35/50 kDa mucins, the major surface glycoproteins from T. cruzi insect-dwelling forms, are involved in parasite attachment to the internal cuticle of the triatomine rectal ampoule, a critical step leading to its differentiation into mammal-infective forms. Experimental evidence supporting this conclusion could be summarized as follows: i) native and recombinant Gp35/50 kDa mucins directly interacted with hindgut tissues from Triatoma infestans, as assessed by indirect immunofluorescence assays; ii) transgenic epimastigotes over-expressing Gp35/50 kDa mucins on their surface coat exhibited improved attachment rates (~2-3 fold) to such tissues as compared to appropriate transgenic controls and/or wild-type counterparts; and iii) certain chemically synthesized compounds derived from Gp35/50 kDa mucins were able to specifically interfere with epimastigote attachment to the inner lining of T. infestans rectal ampoules in ex vivo binding assays, most likely by competing with or directly blocking insect receptor(s). A solvent-exposed peptide (smugS peptide) from the Gp35/50 kDa mucins protein scaffolds and a branched, Galf-containing trisaccharide (Galfβ1-4[Galpβ1-6]GlcNAcα) from their O-linked glycans were identified as main adhesion determinants for these molecules. Interestingly, exogenous addition of a synthetic Galfβ1-4[Galpβ1-6]GlcNAcα derivative or of oligosaccharides containing this structure impaired the attachment of Dm28c but not of CL Brener epimastigotes to triatomine hindgut tissues; which correlates with the presence of Galf residues on the Gp35/50 kDa mucins' O-glycans on the former but not the latter parasite clone.
Conclusion/significance: These results provide novel insights into the mechanisms underlying T. cruzi-triatomine interplay, and indicate that inter-strain variations in the O-glycosylation of Gp35/50 kDa mucins may lead to differences in parasite differentiation and hence, in parasite transmissibility to the mammalian host. Most importantly, our findings point to Gp35/50 kDa mucins and/or the Galf biosynthetic pathway, which is absent in mammals and insects, as appealing targets for the development of T. cruzi transmission-blocking strategies.
Conflict of interest statement
The authors have declared that no competing interests exist
Figures
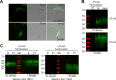
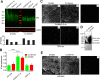
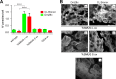
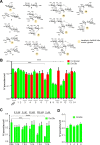
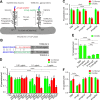
Similar articles
-
Gp35/50 mucin molecules of Trypanosoma cruzi metacyclic forms that mediate host cell invasion interact with annexin A2.PLoS Negl Trop Dis. 2022 Oct 3;16(10):e0010788. doi: 10.1371/journal.pntd.0010788. eCollection 2022 Oct. PLoS Negl Trop Dis. 2022. PMID: 36190932 Free PMC article.
-
Trypanosoma cruzi TcSMUG L-surface mucins promote development and infectivity in the triatomine vector Rhodnius prolixus.PLoS Negl Trop Dis. 2013 Nov 14;7(11):e2552. doi: 10.1371/journal.pntd.0002552. eCollection 2013 Nov. PLoS Negl Trop Dis. 2013. PMID: 24244781 Free PMC article.
-
Addition of α-O-GlcNAc to threonine residues define the post-translational modification of mucin-like molecules in Trypanosoma cruzi.Glycoconj J. 2013 Oct;30(7):659-66. doi: 10.1007/s10719-013-9469-7. Epub 2013 Feb 21. Glycoconj J. 2013. PMID: 23430107 Free PMC article. Review.
-
Comparing vector competence of Mepraia gajardoi and Triatoma infestans by genotyping Trypanosoma cruzi discrete typing units present in naturally infected Octodon degus.Acta Trop. 2019 Feb;190:119-122. doi: 10.1016/j.actatropica.2018.11.007. Epub 2018 Nov 12. Acta Trop. 2019. PMID: 30439345
-
What makes an effective Chagas disease vector? Factors underlying Trypanosoma cruzi-triatomine interactions.Acta Trop. 2018 Jul;183:23-31. doi: 10.1016/j.actatropica.2018.04.008. Epub 2018 Apr 3. Acta Trop. 2018. PMID: 29625091 Review.
Cited by
-
The End Justifies the Means: Chagas Disease from a Perspective of the Host-Trypanosoma cruzi Interaction.Life (Basel). 2024 Apr 9;14(4):488. doi: 10.3390/life14040488. Life (Basel). 2024. PMID: 38672758 Free PMC article. Review.
-
Trypanosoma Cruzi Genome: Organization, Multi-Gene Families, Transcription, and Biological Implications.Genes (Basel). 2020 Oct 14;11(10):1196. doi: 10.3390/genes11101196. Genes (Basel). 2020. PMID: 33066599 Free PMC article. Review.
-
Interaction of Trypanosoma cruzi, Triatomines and the Microbiota of the Vectors-A Review.Microorganisms. 2024 Apr 25;12(5):855. doi: 10.3390/microorganisms12050855. Microorganisms. 2024. PMID: 38792688 Free PMC article. Review.
-
A sticky situation: When trypanosomatids attach to insect tissues.PLoS Pathog. 2023 Dec 21;19(12):e1011854. doi: 10.1371/journal.ppat.1011854. eCollection 2023 Dec. PLoS Pathog. 2023. PMID: 38128049 Free PMC article. Review.
-
Trypanosoma cruzi genetic diversity: impact on transmission cycles and Chagas disease.Mem Inst Oswaldo Cruz. 2022 May 6;117:e210193. doi: 10.1590/0074-02760210193. eCollection 2022. Mem Inst Oswaldo Cruz. 2022. PMID: 35544857 Free PMC article.
References
-
- Kollien AH, Schaub GA (2000) The development of Trypanosoma cruzi in triatominae. Parasitol Today 16: 381–387. - PubMed
Publication types
MeSH terms
Substances
LinkOut - more resources
Full Text Sources