Smoothened stimulation by membrane sterols drives Hedgehog pathway activity
- PMID: 31263273
- PMCID: PMC6709672
- DOI: 10.1038/s41586-019-1355-4
Smoothened stimulation by membrane sterols drives Hedgehog pathway activity
Abstract
Hedgehog signalling is fundamental to embryonic development and postnatal tissue regeneration1. Aberrant postnatal Hedgehog signalling leads to several malignancies, including basal cell carcinoma and paediatric medulloblastoma2. Hedgehog proteins bind to and inhibit the transmembrane cholesterol transporter Patched-1 (PTCH1), which permits activation of the seven-transmembrane transducer Smoothened (SMO) via a mechanism that is poorly understood. Here we report the crystal structure of active mouse SMO bound to both the agonist SAG21k and to an intracellular binding nanobody that stabilizes a physiologically relevant active state. Analogous to other G protein-coupled receptors, the activation of SMO is associated with subtle motions in the extracellular domain, and larger intracellular changes. In contrast to recent models3-5, a cholesterol molecule that is critical for SMO activation is bound deep within the seven-transmembrane pocket. We propose that the inactivation of PTCH1 by Hedgehog allows a transmembrane sterol to access this seven-transmembrane site (potentially through a hydrophobic tunnel), which drives the activation of SMO. These results-combined with signalling studies and molecular dynamics simulations-delineate the structural basis for PTCH1-SMO regulation, and suggest a strategy for overcoming clinical resistance to SMO inhibitors.
Figures
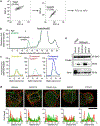
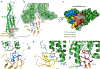
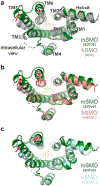
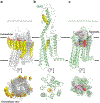
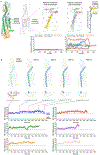
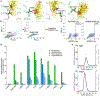
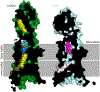
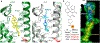
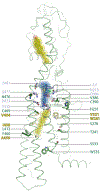
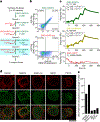
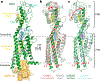
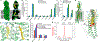
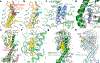
Similar articles
-
Structural Basis of Smoothened Activation in Hedgehog Signaling.Cell. 2018 Jul 12;174(2):312-324.e16. doi: 10.1016/j.cell.2018.04.029. Epub 2018 May 24. Cell. 2018. PMID: 29804838 Free PMC article.
-
A sterol analog inhibits hedgehog pathway by blocking cholesterylation of smoothened.Cell Chem Biol. 2024 Jul 18;31(7):1264-1276.e7. doi: 10.1016/j.chembiol.2024.02.002. Epub 2024 Mar 4. Cell Chem Biol. 2024. PMID: 38442710
-
Sterol regulation of developmental and oncogenic Hedgehog signaling.Biochem Pharmacol. 2022 Feb;196:114647. doi: 10.1016/j.bcp.2021.114647. Epub 2021 Jun 7. Biochem Pharmacol. 2022. PMID: 34111427 Free PMC article. Review.
-
Sterols in an intramolecular channel of Smoothened mediate Hedgehog signaling.Nat Chem Biol. 2020 Dec;16(12):1368-1375. doi: 10.1038/s41589-020-0646-2. Epub 2020 Sep 14. Nat Chem Biol. 2020. PMID: 32929279 Free PMC article.
-
Cholesterol access in cellular membranes controls Hedgehog signaling.Nat Chem Biol. 2020 Dec;16(12):1303-1313. doi: 10.1038/s41589-020-00678-2. Epub 2020 Nov 16. Nat Chem Biol. 2020. PMID: 33199907 Free PMC article. Review.
Cited by
-
Pathological implications of metabolic reprogramming and its therapeutic potential in medulloblastoma.Front Cell Dev Biol. 2022 Oct 19;10:1007641. doi: 10.3389/fcell.2022.1007641. eCollection 2022. Front Cell Dev Biol. 2022. PMID: 36340043 Free PMC article. Review.
-
Accelerating GPCR Drug Discovery With Conformation-Stabilizing VHHs.Front Mol Biosci. 2022 May 23;9:863099. doi: 10.3389/fmolb.2022.863099. eCollection 2022. Front Mol Biosci. 2022. PMID: 35677880 Free PMC article. Review.
-
Frizzled BRET sensors based on bioorthogonal labeling of unnatural amino acids reveal WNT-induced dynamics of the cysteine-rich domain.Sci Adv. 2021 Nov 12;7(46):eabj7917. doi: 10.1126/sciadv.abj7917. Epub 2021 Nov 10. Sci Adv. 2021. PMID: 34757789 Free PMC article.
-
Notum Deletion From Late-Stage Skeletal Cells Increases Cortical Bone Formation and Potentiates Skeletal Effects of Sclerostin Inhibition.J Bone Miner Res. 2021 Dec;36(12):2413-2425. doi: 10.1002/jbmr.4411. Epub 2021 Jul 23. J Bone Miner Res. 2021. PMID: 34223673 Free PMC article.
-
The Inseparable Relationship Between Cholesterol and Hedgehog Signaling.Annu Rev Biochem. 2023 Jun 20;92:273-298. doi: 10.1146/annurev-biochem-052521-040313. Epub 2023 Mar 31. Annu Rev Biochem. 2023. PMID: 37001135 Free PMC article. Review.
References
-
- Briscoe J & Thérond PP The mechanisms of Hedgehog signalling and its roles in development and disease. Nat. Rev. Mol. Cell Biol. 14, 416–429 (2013). - PubMed
MeSH terms
Substances
Grants and funding
LinkOut - more resources
Full Text Sources
Other Literature Sources
Molecular Biology Databases
Miscellaneous