Genomes of early-diverging streptophyte algae shed light on plant terrestrialization
- PMID: 31844283
- PMCID: PMC7027972
- DOI: 10.1038/s41477-019-0560-3
Genomes of early-diverging streptophyte algae shed light on plant terrestrialization
Abstract
Mounting evidence suggests that terrestrialization of plants started in streptophyte green algae, favoured by their dual existence in freshwater and subaerial/terrestrial environments. Here, we present the genomes of Mesostigma viride and Chlorokybus atmophyticus, two sister taxa in the earliest-diverging clade of streptophyte algae dwelling in freshwater and subaerial/terrestrial environments, respectively. We provide evidence that the common ancestor of M. viride and C. atmophyticus (and thus of streptophytes) had already developed traits associated with a subaerial/terrestrial environment, such as embryophyte-type photorespiration, canonical plant phytochrome, several phytohormones and transcription factors involved in responses to environmental stresses, and evolution of cellulose synthase and cellulose synthase-like genes characteristic of embryophytes. Both genomes differed markedly in genome size and structure, and in gene family composition, revealing their dynamic nature, presumably in response to adaptations to their contrasting environments. The ancestor of M. viride possibly lost several genomic traits associated with a subaerial/terrestrial environment following transition to a freshwater habitat.
Conflict of interest statement
The authors declare no competing interests.
Figures
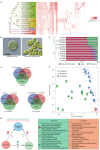
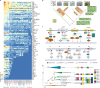
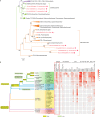
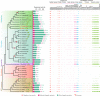
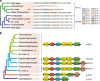
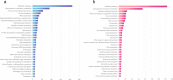
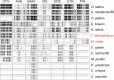
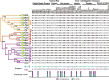
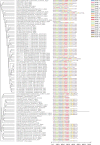
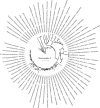
Similar articles
-
Streptophyte algae and the origin of embryophytes.Ann Bot. 2009 May;103(7):999-1004. doi: 10.1093/aob/mcp044. Epub 2009 Mar 8. Ann Bot. 2009. PMID: 19273476 Free PMC article.
-
A clade uniting the green algae Mesostigma viride and Chlorokybus atmophyticus represents the deepest branch of the Streptophyta in chloroplast genome-based phylogenies.BMC Biol. 2007 Jan 12;5:2. doi: 10.1186/1741-7007-5-2. BMC Biol. 2007. PMID: 17222354 Free PMC article.
-
Genomes of Subaerial Zygnematophyceae Provide Insights into Land Plant Evolution.Cell. 2019 Nov 14;179(5):1057-1067.e14. doi: 10.1016/j.cell.2019.10.019. Cell. 2019. PMID: 31730849
-
Tracking the evolutionary innovations of plant terrestrialization.Gene. 2021 Feb 15;769:145203. doi: 10.1016/j.gene.2020.145203. Epub 2020 Oct 5. Gene. 2021. PMID: 33031891 Review.
-
Draparnaldia: a chlorophyte model for comparative analyses of plant terrestrialization.J Exp Bot. 2020 Jun 11;71(11):3305-3313. doi: 10.1093/jxb/eraa102. J Exp Bot. 2020. PMID: 32100007 Review.
Cited by
-
A Genomic Perspective on the Evolutionary Diversity of the Plant Cell Wall.Plants (Basel). 2020 Sep 12;9(9):1195. doi: 10.3390/plants9091195. Plants (Basel). 2020. PMID: 32932717 Free PMC article. Review.
-
Genome-wide analyses across Viridiplantae reveal the origin and diversification of small RNA pathway-related genes.Commun Biol. 2021 Mar 25;4(1):412. doi: 10.1038/s42003-021-01933-5. Commun Biol. 2021. PMID: 33767367 Free PMC article.
-
Evolutionary Trends in the Mitochondrial Genome of Archaeplastida: How Does the GC Bias Affect the Transition from Water to Land?Plants (Basel). 2020 Mar 12;9(3):358. doi: 10.3390/plants9030358. Plants (Basel). 2020. PMID: 32178249 Free PMC article.
-
Desiccation tolerance in streptophyte algae and the algae to land plant transition: evolution of LEA and MIP protein families within the Viridiplantae.J Exp Bot. 2020 Jun 11;71(11):3270-3278. doi: 10.1093/jxb/eraa105. J Exp Bot. 2020. PMID: 32107542 Free PMC article. Review.
-
A Comprehensive Phylogenetic Analysis of the MAP4K Family in the Green Lineage.Front Plant Sci. 2021 Aug 13;12:650171. doi: 10.3389/fpls.2021.650171. eCollection 2021. Front Plant Sci. 2021. PMID: 34484252 Free PMC article.
References
-
- Kenrick P, Crane P. The origin and early evolution of plants on land. Nature. 1997;389:33–39.
-
- Delwiche CF, Cooper ED. The evolutionary origin of a terrestrial flora. Curr. Biol. 2015;25:R899–R910. - PubMed
-
- Bowman JL, et al. Insights into land plant evolution garnered from the Marchantia polymorpha genome. Cell. 2017;171:287–304. - PubMed
Publication types
MeSH terms
LinkOut - more resources
Full Text Sources