The genome of Prasinoderma coloniale unveils the existence of a third phylum within green plants
- PMID: 32572216
- PMCID: PMC7455551
- DOI: 10.1038/s41559-020-1221-7
The genome of Prasinoderma coloniale unveils the existence of a third phylum within green plants
Erratum in
-
Author Correction: The genome of Prasinoderma coloniale unveils the existence of a third phylum within green plants.Nat Ecol Evol. 2020 Sep;4(9):1280. doi: 10.1038/s41559-020-1268-5. Nat Ecol Evol. 2020. PMID: 32678322 Free PMC article.
Abstract
Genome analysis of the pico-eukaryotic marine green alga Prasinoderma coloniale CCMP 1413 unveils the existence of a novel phylum within green plants (Viridiplantae), the Prasinodermophyta, which diverged before the split of Chlorophyta and Streptophyta. Structural features of the genome and gene family comparisons revealed an intermediate position of the P. coloniale genome (25.3 Mb) between the extremely compact, small genomes of picoplanktonic Mamiellophyceae (Chlorophyta) and the larger, more complex genomes of early-diverging streptophyte algae. Reconstruction of the minimal core genome of Viridiplantae allowed identification of an ancestral toolkit of transcription factors and flagellar proteins. Adaptations of P. coloniale to its deep-water, oligotrophic environment involved expansion of light-harvesting proteins, reduction of early light-induced proteins, evolution of a distinct type of C4 photosynthesis and carbon-concentrating mechanism, synthesis of the metal-complexing metabolite picolinic acid, and vitamin B1, B7 and B12 auxotrophy. The P. coloniale genome provides first insights into the dawn of green plant evolution.
Conflict of interest statement
The authors declare no competing interests.
Figures
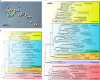
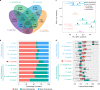
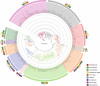
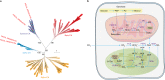
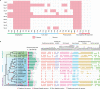
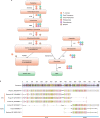
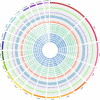
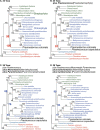
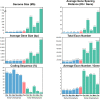
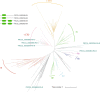
Comment in
-
A planktonic picoeukaryote makes big changes to the green lineage.Nat Ecol Evol. 2020 Sep;4(9):1160-1161. doi: 10.1038/s41559-020-1244-0. Nat Ecol Evol. 2020. PMID: 32591748 No abstract available.
Similar articles
-
Six newly sequenced chloroplast genomes from prasinophyte green algae provide insights into the relationships among prasinophyte lineages and the diversity of streamlined genome architecture in picoplanktonic species.BMC Genomics. 2014 Oct 4;15(1):857. doi: 10.1186/1471-2164-15-857. BMC Genomics. 2014. PMID: 25281016 Free PMC article.
-
Complete mitogenomes of the marine picoplanktonic green algae Prasinoderma sp. MBIC 10622 and Prasinococcus capsulatus CCMP 1194 (Palmophyllophyceae).Mitochondrial DNA B Resour. 2019 Dec 11;5(1):166-168. doi: 10.1080/23802359.2019.1698370. Mitochondrial DNA B Resour. 2019. PMID: 33366470 Free PMC article.
-
The complete mitochondrial DNA sequence of Mesostigma viride identifies this green alga as the earliest green plant divergence and predicts a highly compact mitochondrial genome in the ancestor of all green plants.Mol Biol Evol. 2002 Jan;19(1):24-38. doi: 10.1093/oxfordjournals.molbev.a003979. Mol Biol Evol. 2002. PMID: 11752187
-
Into the deep: new discoveries at the base of the green plant phylogeny.Bioessays. 2011 Sep;33(9):683-92. doi: 10.1002/bies.201100035. Epub 2011 Jul 11. Bioessays. 2011. PMID: 21744372 Review.
-
The Land-Sea Connection: Insights Into the Plant Lineage from a Green Algal Perspective.Annu Rev Plant Biol. 2022 May 20;73:585-616. doi: 10.1146/annurev-arplant-071921-100530. Epub 2022 Mar 8. Annu Rev Plant Biol. 2022. PMID: 35259927 Review.
Cited by
-
Phytoplankton Surveys in the Arctic Fram Strait Demonstrate the Tiny Eukaryotic Alga Micromonas and Other Picoprasinophytes Contribute to Deep Sea Export.Microorganisms. 2022 May 3;10(5):961. doi: 10.3390/microorganisms10050961. Microorganisms. 2022. PMID: 35630405 Free PMC article.
-
Genomic characterisation and ecological distribution of Mantoniella tinhauana: a novel Mamiellophycean green alga from the Western Pacific.Front Microbiol. 2024 May 7;15:1358574. doi: 10.3389/fmicb.2024.1358574. eCollection 2024. Front Microbiol. 2024. PMID: 38774501 Free PMC article.
-
Presence of vitamin B12 metabolism in the last common ancestor of land plants.Philos Trans R Soc Lond B Biol Sci. 2024 Nov 18;379(1914):20230354. doi: 10.1098/rstb.2023.0354. Epub 2024 Sep 30. Philos Trans R Soc Lond B Biol Sci. 2024. PMID: 39343018 Free PMC article.
-
MarFERReT, an open-source, version-controlled reference library of marine microbial eukaryote functional genes.Sci Data. 2023 Dec 21;10(1):926. doi: 10.1038/s41597-023-02842-4. Sci Data. 2023. PMID: 38129449 Free PMC article.
-
Genome-wide analyses across Viridiplantae reveal the origin and diversification of small RNA pathway-related genes.Commun Biol. 2021 Mar 25;4(1):412. doi: 10.1038/s42003-021-01933-5. Commun Biol. 2021. PMID: 33767367 Free PMC article.
References
-
- Niklas, K. J. The Evolutionary Biology of Plants (Univ. of Chicago Press, 1997).
-
- Kenrick P, Crane P. The origin and early evolution of plants on Land. Nature. 1997;389:33–39.
-
- Willis, K. & McElwain, J. The Evolution of Plants (Oxford Univ. Press, 2014).
-
- Judd, W. S., Campbell, C. S., Kellogg, E. A., Stevens, P. F. & Donoghue, M. J. Plant Systematics: A Phylogenetic Approach (Sinauer, 2008).
-
- Courties C, et al. Smallest eukaryotic organism. Nature. 1994;370:255.
Publication types
MeSH terms
LinkOut - more resources
Full Text Sources
Miscellaneous