RNA-seq analysis of galaninergic neurons from ventrolateral preoptic nucleus identifies expression changes between sleep and wake
- PMID: 32928100
- PMCID: PMC7491139
- DOI: 10.1186/s12864-020-07050-7
RNA-seq analysis of galaninergic neurons from ventrolateral preoptic nucleus identifies expression changes between sleep and wake
Abstract
Background: Previous studies show that galanin neurons in ventrolateral preoptic nucleus (VLPO-Gal) are essential for sleep regulation. Here, we explored the transcriptional regulation of the VLPO-Gal neurons in sleep by comparing their transcriptional responses between sleeping mice and those kept awake, sacrificed at the same diurnal time.
Results: RNA-sequencing (RNA-seq) analysis was performed on eGFP(+) galanin neurons isolated using laser captured microdissection (LCM) from VLPO. Expression of Gal was assessed in our LCM eGFP(+) neurons via real time qPCR and showed marked enrichment when compared to LCM eGFP(-) cells and to bulk VLPO samples. Gene set enrichment analysis utilizing data from a recent single-cell RNA-seq study of the preoptic area demonstrated that our VLPO-Gal samples were highly enriched with galanin-expressing inhibitory neurons, but not galanin-expressing excitatory neurons. A total of 263 genes were differentially expressed between sleep and wake in VLPO-Gal neurons. When comparing differentially expressed genes in VLPO-Gal neurons to differentially expressed genes in a wake-active neuronal region (the medial prefrontal cortex), evidence indicates that both systemic and cell-specific mechanisms contribute to the transcriptional regulation in VLPO-Gal neurons. In both wake-active and sleep-active neurons, ER stress pathways are activated by wake and cold-inducible RNA-binding proteins are activated by sleep. In contrast, expression of DNA repair genes is increased in VLPO-Gal during wakefulness, but increased in wake-active cells during sleep.
Conclusion: Our study identified transcriptomic responses of the galanin neurons in the ventrolateral preoptic nucleus during sleep and sleep deprivation. Data indicate that VLPO contains mainly sleep-active inhibitory galaninergic neurons. The VLPO galanin neurons show responses to sleep and wake similar to wake-active regions, indicating these responses, such as ER stress and cold-inducible RNA-binding proteins, are systemic affecting all neuronal populations. Region-specific differences in sleep/wake responses were also identified, in particular DNA repair. Our study expands knowledge about the transcriptional response of a distinct group of neurons essential for sleep.
Keywords: Galaninergic neurons; Next-generation RNA-sequencing; Sleep deprivation; Spontaneous sleep; Ventrolateral preoptic nucleus.
Conflict of interest statement
The authors declare that they have no competing interests.
Figures
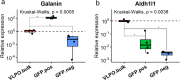
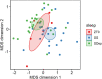
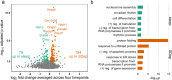
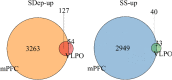
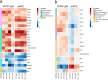
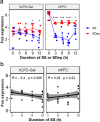
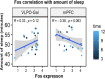
Similar articles
-
Structure and Function of Neuronal Circuits Linking Ventrolateral Preoptic Nucleus and Lateral Hypothalamic Area.J Neurosci. 2023 May 31;43(22):4075-4092. doi: 10.1523/JNEUROSCI.1913-22.2023. Epub 2023 Apr 28. J Neurosci. 2023. PMID: 37117013 Free PMC article.
-
Galanin neurons in the ventrolateral preoptic area promote sleep and heat loss in mice.Nat Commun. 2018 Oct 8;9(1):4129. doi: 10.1038/s41467-018-06590-7. Nat Commun. 2018. PMID: 30297727 Free PMC article.
-
Ventrolateral preoptic nucleus contains sleep-active, galaninergic neurons in multiple mammalian species.Neuroscience. 2002;115(1):285-94. doi: 10.1016/s0306-4522(02)00308-1. Neuroscience. 2002. PMID: 12401341
-
The Sleep-Promoting Ventrolateral Preoptic Nucleus: What Have We Learned over the Past 25 Years?Int J Mol Sci. 2022 Mar 8;23(6):2905. doi: 10.3390/ijms23062905. Int J Mol Sci. 2022. PMID: 35328326 Free PMC article. Review.
-
Underlying brain mechanisms that regulate sleep-wakefulness cycles.Int Rev Neurobiol. 2010;93:1-21. doi: 10.1016/S0074-7742(10)93001-8. Int Rev Neurobiol. 2010. PMID: 20969999 Review.
Cited by
-
Probing pathways by which rhynchophylline modifies sleep using spatial transcriptomics.Biol Direct. 2023 May 5;18(1):21. doi: 10.1186/s13062-023-00377-7. Biol Direct. 2023. PMID: 37143153 Free PMC article.
-
QPLOT Neurons-Converging on a Thermoregulatory Preoptic Neuronal Population.Front Neurosci. 2021 May 4;15:665762. doi: 10.3389/fnins.2021.665762. eCollection 2021. Front Neurosci. 2021. PMID: 34017237 Free PMC article.
-
Single-nucleus RNA-seq identifies one galanin neuronal subtype in mouse preoptic hypothalamus activated during recovery from sleep deprivation.Cell Rep. 2024 May 28;43(5):114192. doi: 10.1016/j.celrep.2024.114192. Epub 2024 May 3. Cell Rep. 2024. PMID: 38703367 Free PMC article.
-
Sleep Disorders: Pathogenesis and Therapeutic Interventions.MedComm (2020). 2025 Mar 10;6(3):e70130. doi: 10.1002/mco2.70130. eCollection 2025 Mar. MedComm (2020). 2025. PMID: 40066230 Free PMC article. Review.
References
-
- Acosta-Alvear D, Zhou Y, Blais A, Tsikitis M, Lents NH, Arias C, Lennon CJ, Kluger Y, Dynlacht BD. XBP1 controls diverse cell type- and condition-specific transcriptional regulatory networks. Mol Cell. 2007;27(1):53–66. - PubMed
-
- Bekker-Jensen S, Rendtlew Danielsen J, Fugger K, Gromova I, Nerstedt A, Lukas C, Bartek J, Lukas J, Mailand N. HERC2 coordinates ubiquitin-dependent assembly of DNA repair factors on damaged chromosomes. Nat Cell Biol. 2010;12(1):80–86. - PubMed
-
- Benjamini Y, Hochberg Y. Controlling the false discovery rate - a practical and powerful approach to multiple testing. J Royal Stat Soc Series B-Methodol. 1995;57(1):289–300.
MeSH terms
Substances
Grants and funding
LinkOut - more resources
Full Text Sources
Molecular Biology Databases