Platypus and echidna genomes reveal mammalian biology and evolution
- PMID: 33408411
- PMCID: PMC8081666
- DOI: 10.1038/s41586-020-03039-0
Platypus and echidna genomes reveal mammalian biology and evolution
Abstract
Egg-laying mammals (monotremes) are the only extant mammalian outgroup to therians (marsupial and eutherian animals) and provide key insights into mammalian evolution1,2. Here we generate and analyse reference genomes of the platypus (Ornithorhynchus anatinus) and echidna (Tachyglossus aculeatus), which represent the only two extant monotreme lineages. The nearly complete platypus genome assembly has anchored almost the entire genome onto chromosomes, markedly improving the genome continuity and gene annotation. Together with our echidna sequence, the genomes of the two species allow us to detect the ancestral and lineage-specific genomic changes that shape both monotreme and mammalian evolution. We provide evidence that the monotreme sex chromosome complex originated from an ancestral chromosome ring configuration. The formation of such a unique chromosome complex may have been facilitated by the unusually extensive interactions between the multi-X and multi-Y chromosomes that are shared by the autosomal homologues in humans. Further comparative genomic analyses unravel marked differences between monotremes and therians in haptoglobin genes, lactation genes and chemosensory receptor genes for smell and taste that underlie the ecological adaptation of monotremes.
Conflict of interest statement
J.K. is an employee of Pacific Biosciences, a company that develops single-molecule sequencing technologies.
Figures
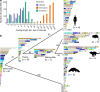
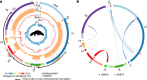
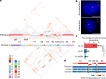
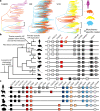
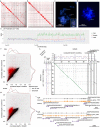
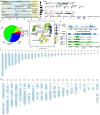
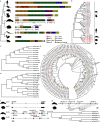
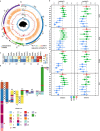
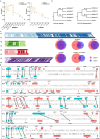
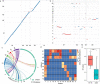
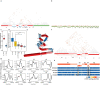
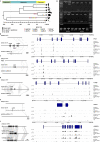
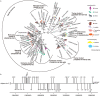
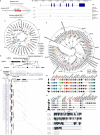
Similar articles
-
Comparative genomics of monotremes provides insights into the early evolution of mammalian epidermal differentiation genes.Sci Rep. 2024 Jan 16;14(1):1437. doi: 10.1038/s41598-024-51926-7. Sci Rep. 2024. PMID: 38228724 Free PMC article.
-
Chromosome-level echidna genome illuminates evolution of multiple sex chromosome system in monotremes.Gigascience. 2025 Jan 6;14:giae112. doi: 10.1093/gigascience/giae112. Gigascience. 2025. PMID: 39778707 Free PMC article.
-
Qualitative evidence synthesis informing our understanding of people's perceptions and experiences of targeted digital communication.Cochrane Database Syst Rev. 2019 Oct 23;10(10):ED000141. doi: 10.1002/14651858.ED000141. Cochrane Database Syst Rev. 2019. PMID: 31643081 Free PMC article.
-
Trends in Surgical and Nonsurgical Aesthetic Procedures: A 14-Year Analysis of the International Society of Aesthetic Plastic Surgery-ISAPS.Aesthetic Plast Surg. 2024 Oct;48(20):4217-4227. doi: 10.1007/s00266-024-04260-2. Epub 2024 Aug 5. Aesthetic Plast Surg. 2024. PMID: 39103642 Review.
-
Harnessing Biological Insight to Accelerate Tuberculosis Drug Discovery.Acc Chem Res. 2019 Aug 20;52(8):2340-2348. doi: 10.1021/acs.accounts.9b00275. Epub 2019 Jul 30. Acc Chem Res. 2019. PMID: 31361123 Free PMC article. Review.
Cited by
-
Monotreme-specific conserved putative proteins derived from retroviral reverse transcriptase.Virus Evol. 2022 Sep 3;8(2):veac084. doi: 10.1093/ve/veac084. eCollection 2022. Virus Evol. 2022. PMID: 36176487 Free PMC article.
-
A brief review of vertebrate sex evolution with a pledge for integrative research: towards 'sexomics'.Philos Trans R Soc Lond B Biol Sci. 2021 Aug 30;376(1832):20200426. doi: 10.1098/rstb.2020.0426. Epub 2021 Jul 12. Philos Trans R Soc Lond B Biol Sci. 2021. PMID: 34247497 Free PMC article. Review.
-
A family of olfactory receptors uniquely expanded in marsupial and monotreme genomes are expressed by a T cell subset also unique to marsupials and monotremes.Dev Comp Immunol. 2024 May;154:105149. doi: 10.1016/j.dci.2024.105149. Epub 2024 Feb 8. Dev Comp Immunol. 2024. PMID: 38340883 Free PMC article.
-
Functional Diversity and Evolution of Bitter Taste Receptors in Egg-Laying Mammals.Mol Biol Evol. 2022 Jun 2;39(6):msac107. doi: 10.1093/molbev/msac107. Mol Biol Evol. 2022. PMID: 35652727 Free PMC article.
-
Dynamic Evolution of Retroviral Envelope Genes in Egg-Laying Mammalian Genomes.Mol Biol Evol. 2023 May 2;40(5):msad090. doi: 10.1093/molbev/msad090. Mol Biol Evol. 2023. PMID: 37062963 Free PMC article.
References
-
- Ashwell, K. Neurobiology of Monotremes: Brain Evolution in Our Distant Mammalian Cousins (CSIRO PUBLISHING, 2013).
-
- Grützner F, et al. In the platypus a meiotic chain of ten sex chromosomes shares genes with the bird Z and mammal X chromosomes. Nature. 2004;432:913–917. - PubMed
-
- Kortschak RD, Tsend-Ayush E, Grützner F. Analysis of SINE and LINE repeat content of Y chromosomes in the platypus, Ornithorhynchus anatinus. Reprod. Fertil. Dev. 2009;21:964–975. - PubMed
Publication types
MeSH terms
Grants and funding
LinkOut - more resources
Full Text Sources
Other Literature Sources