Trophic flexibility of marine diplonemids - switching from osmotrophy to bacterivory
- PMID: 35042972
- PMCID: PMC9039065
- DOI: 10.1038/s41396-022-01192-0
Trophic flexibility of marine diplonemids - switching from osmotrophy to bacterivory
Abstract
Diplonemids are one of the most abundant groups of heterotrophic planktonic microeukaryotes in the world ocean and, thus, are likely to play an essential role in marine ecosystems. So far, only few species have been introduced into a culture, allowing basic studies of diplonemid genetics, morphology, ultrastructure, metabolism, as well as endosymbionts. However, it remains unclear whether these heterotrophic flagellates are parasitic or free-living and what are their predominant dietary patterns and preferred food items. Here we show that cultured diplonemids, maintained in an organic-rich medium as osmotrophs, can gradually switch to bacterivory as a sole food resource, supporting positive growth of their population, even when fed with a low biovolume of bacteria. We further observed remarkable differences in species-specific feeding patterns, size-selective grazing preferences, and distinct feeding strategies. Diplonemids can discriminate between low-quality food items and inedible particles, such as latex beads, even after their ingestion, by discharging them in the form of large waste vacuoles. We also detected digestion-related endogenous autofluorescence emitted by lysosomes and the activity of a melanin-like material. We present the first evidence that these omnipresent protists possess an opportunistic lifestyle that provides a considerable advantage in the generally food resource-limited marine environments.
© 2022. The Author(s), under exclusive licence to International Society for Microbial Ecology.
Conflict of interest statement
The authors declare no competing interests.
Figures
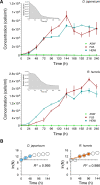
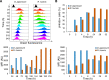
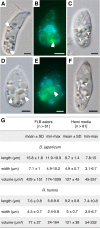
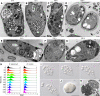
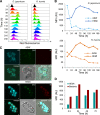
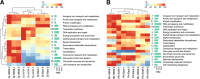
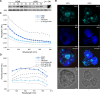
Similar articles
-
Massive Accumulation of Strontium and Barium in Diplonemid Protists.mBio. 2023 Feb 28;14(1):e0327922. doi: 10.1128/mbio.03279-22. Epub 2023 Jan 16. mBio. 2023. PMID: 36645306 Free PMC article.
-
Extreme Diversity of Diplonemid Eukaryotes in the Ocean.Curr Biol. 2016 Nov 21;26(22):3060-3065. doi: 10.1016/j.cub.2016.09.031. Curr Biol. 2016. PMID: 27875689
-
Bacterivory by heterotrophic flagellates: community structure and feeding strategies.Antonie Van Leeuwenhoek. 2002 Aug;81(1-4):465-80. doi: 10.1023/a:1020509305868. Antonie Van Leeuwenhoek. 2002. PMID: 12448743 Review.
-
Confusing selective feeding with differential digestion in bacterivorous nanoflagellates.J Eukaryot Microbiol. 2001 Jul-Aug;48(4):425-32. doi: 10.1111/j.1550-7408.2001.tb00175.x. J Eukaryot Microbiol. 2001. PMID: 11456318
-
Diplonemids - A Review on "New" Flagellates on the Oceanic Block.Protist. 2022 Apr;173(2):125868. doi: 10.1016/j.protis.2022.125868. Epub 2022 Mar 3. Protist. 2022. PMID: 35339983 Review.
Cited by
-
Recent expansion of metabolic versatility in Diplonema papillatum, the model species of a highly speciose group of marine eukaryotes.BMC Biol. 2023 May 4;21(1):99. doi: 10.1186/s12915-023-01563-9. BMC Biol. 2023. PMID: 37143068 Free PMC article.
-
Gene expression dynamics of natural assemblages of heterotrophic flagellates during bacterivory.Microbiome. 2023 Jun 15;11(1):134. doi: 10.1186/s40168-023-01571-5. Microbiome. 2023. PMID: 37322519 Free PMC article.
-
Water masses shape pico-nano eukaryotic communities of the Weddell Sea.Commun Biol. 2023 Jan 18;6(1):64. doi: 10.1038/s42003-023-04452-7. Commun Biol. 2023. PMID: 36653511 Free PMC article.
-
Functional differentiation of Sec13 paralogues in the euglenozoan protists.Open Biol. 2023 Jun;13(6):220364. doi: 10.1098/rsob.220364. Epub 2023 Jun 14. Open Biol. 2023. PMID: 37311539 Free PMC article.
-
Massive Accumulation of Strontium and Barium in Diplonemid Protists.mBio. 2023 Feb 28;14(1):e0327922. doi: 10.1128/mbio.03279-22. Epub 2023 Jan 16. mBio. 2023. PMID: 36645306 Free PMC article.
References
-
- Di Poi E, Blason C, Corinaldesi C, Danovaro R, Malisana E, Fonda-Umani S. Structure and interactions within the pelagic microbial food web (from viruses to microplankton) across environmental gradients in the Mediterranean Sea. Glob Biogeochem Cycles. 2013;27:1034–45. doi: 10.1002/2013GB004589. - DOI
-
- Das S, Mangwani N. Ocean acidification and marine microorganisms: responses and consequences. Oceanologia. 2015;57:349–61. doi: 10.1016/j.oceano.2015.07.003. - DOI