An Enigmatic Stramenopile Sheds Light on Early Evolution in Ochrophyta Plastid Organellogenesis
- PMID: 35348760
- PMCID: PMC9004409
- DOI: 10.1093/molbev/msac065
An Enigmatic Stramenopile Sheds Light on Early Evolution in Ochrophyta Plastid Organellogenesis
Abstract
Ochrophyta is an algal group belonging to the Stramenopiles and comprises diverse lineages of algae which contribute significantly to the oceanic ecosystems as primary producers. However, early evolution of the plastid organelle in Ochrophyta is not fully understood. In this study, we provide a well-supported tree of the Stramenopiles inferred by the large-scale phylogenomic analysis that unveils the eukaryvorous (nonphotosynthetic) protist Actinophrys sol (Actinophryidae) is closely related to Ochrophyta. We used genomic and transcriptomic data generated from A. sol to detect molecular traits of its plastid and we found no evidence of plastid genome and plastid-mediated biosynthesis, consistent with previous ultrastructural studies that did not identify any plastids in Actinophryidae. Moreover, our phylogenetic analyses of particular biosynthetic pathways provide no evidence of a current and past plastid in A. sol. However, we found more than a dozen organellar aminoacyl-tRNA synthases (aaRSs) that are of algal origin. Close relationships between aaRS from A. sol and their ochrophyte homologs document gene transfer of algal genes that happened before the divergence of Actinophryidae and Ochrophyta lineages. We further showed experimentally that organellar aaRSs of A. sol are targeted exclusively to mitochondria, although organellar aaRSs in Ochrophyta are dually targeted to mitochondria and plastids. Together, our findings suggested that the last common ancestor of Actinophryidae and Ochrophyta had not yet completed the establishment of host-plastid partnership as seen in the current Ochrophyta species, but acquired at least certain nuclear-encoded genes for the plastid functions.
Keywords: Actinophryidae; aminoacyl-tRNA synthase; gene transfer; organellar DNA; phylogenomics; plastid evolution.
© The Author(s) 2022. Published by Oxford University Press on behalf of Society for Molecular Biology and Evolution.
Figures
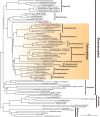
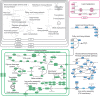
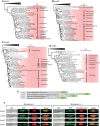
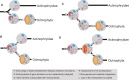
Similar articles
-
Multiple plastid losses within photosynthetic stramenopiles revealed by comprehensive phylogenomics.Curr Biol. 2025 Feb 3;35(3):483-499.e8. doi: 10.1016/j.cub.2024.11.065. Epub 2025 Jan 9. Curr Biol. 2025. PMID: 39793566
-
Updating algal evolutionary relationships through plastid genome sequencing: did alveolate plastids emerge through endosymbiosis of an ochrophyte?Sci Rep. 2015 May 28;5:10134. doi: 10.1038/srep10134. Sci Rep. 2015. PMID: 26017773 Free PMC article.
-
Olisthodiscus represents a new class of Ochrophyta.J Phycol. 2021 Aug;57(4):1094-1118. doi: 10.1111/jpy.13155. Epub 2021 Apr 14. J Phycol. 2021. PMID: 33655496
-
A new scenario of plastid evolution: plastid primary endosymbiosis before the divergence of the "Plantae," emended.J Plant Res. 2005 Aug;118(4):247-55. doi: 10.1007/s10265-005-0219-1. Epub 2005 Jul 20. J Plant Res. 2005. PMID: 16032387 Review.
-
The endosymbiotic origin, diversification and fate of plastids.Philos Trans R Soc Lond B Biol Sci. 2010 Mar 12;365(1541):729-48. doi: 10.1098/rstb.2009.0103. Philos Trans R Soc Lond B Biol Sci. 2010. PMID: 20124341 Free PMC article. Review.
Cited by
-
A cryptic plastid and a novel mitochondrial plasmid in Leucomyxa plasmidifera gen. and sp. nov. (Ochrophyta) push the frontiers of organellar biology.Open Biol. 2024 Oct;14(10):240022. doi: 10.1098/rsob.240022. Epub 2024 Oct 30. Open Biol. 2024. PMID: 39474867 Free PMC article.
-
A Novel Group of Dynamin-Related Proteins Shared by Eukaryotes and Giant Viruses Is Able to Remodel Mitochondria From Within the Matrix.Mol Biol Evol. 2023 Jun 1;40(6):msad134. doi: 10.1093/molbev/msad134. Mol Biol Evol. 2023. PMID: 37279941 Free PMC article.
-
Integrated overview of stramenopile ecology, taxonomy, and heterotrophic origin.ISME J. 2024 Jan 8;18(1):wrae150. doi: 10.1093/ismejo/wrae150. ISME J. 2024. PMID: 39077993 Free PMC article. Review.
-
How marine are Marine Stramenopiles (MAST)? A cross-system evaluation.FEMS Microbiol Ecol. 2024 Oct 25;100(11):fiae130. doi: 10.1093/femsec/fiae130. FEMS Microbiol Ecol. 2024. PMID: 39375832 Free PMC article.
-
Molecular evolution and diversification of phytoene synthase (PSY) gene family.Genet Mol Biol. 2022 Dec 19;45(4):e20210411. doi: 10.1590/1678-4685-GMB-2021-0411. eCollection 2022. Genet Mol Biol. 2022. PMID: 36537743 Free PMC article.
References
-
- Abrahamsen MS, Templeton TJ, Enomoto S, Abrahante JE, Zhu G, Lancto CA, Deng M, Liu C, Widmer G, Tzipori S, et al. . 2004. Complete genome sequence of the apicomplexan, Cryptosporidium parvum. Science 304:441–445. - PubMed
-
- Andersen RA. 2004. Biology and systematics of heterokont and haptophyte algae. Am J Bot. 91:1508–1522. - PubMed
-
- Bringloe TT, Starko S, Wade RM, Vieira C, Kawai H, De Clerck O, Cock JM, Coelho SM, Destombe C, Valero M, et al. . 2020. Phylogeny and evolution of the brown algae. Crit Rev Plant Sci. 39:281–321.
Publication types
MeSH terms
Associated data
LinkOut - more resources
Full Text Sources
Research Materials
Miscellaneous