Biohybrid Micro- and Nanorobots for Intelligent Drug Delivery
- PMID: 36285309
- PMCID: PMC9494704
- DOI: 10.34133/2022/9824057
Biohybrid Micro- and Nanorobots for Intelligent Drug Delivery
Abstract
Biohybrid micro- and nanorobots are integrated tiny machines from biological components and artificial components. They can possess the advantages of onboard actuation, sensing, control, and implementation of multiple medical tasks such as targeted drug delivery, single-cell manipulation, and cell microsurgery. This review paper is to give an overview of biohybrid micro- and nanorobots for smart drug delivery applications. First, a wide range of biohybrid micro- and nanorobots comprising different biological components are reviewed in detail. Subsequently, the applications of biohybrid micro- and nanorobots for active drug delivery are introduced to demonstrate how such biohybrid micro- and nanorobots are being exploited in the field of medicine and healthcare. Lastly, key challenges to be overcome are discussed to pave the way for the clinical translation and application of the biohybrid micro- and nanorobots.
Copyright © 2022 Jinhua Li et al.
Conflict of interest statement
The authors have no conflict of interest or financial ties to disclose.
Figures
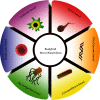
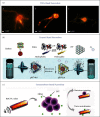
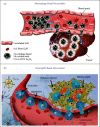
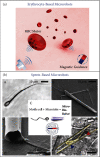
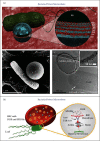
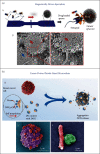
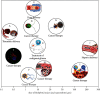
Similar articles
-
Design, fabrication and application of magnetically actuated micro/nanorobots: a review.Nanotechnology. 2022 Jan 18;33(15). doi: 10.1088/1361-6528/ac43e6. Nanotechnology. 2022. PMID: 34915458 Review.
-
Micro-nanorobots: important considerations when developing novel drug delivery platforms.Expert Opin Drug Deliv. 2019 Nov;16(11):1259-1275. doi: 10.1080/17425247.2019.1676228. Epub 2019 Oct 14. Expert Opin Drug Deliv. 2019. PMID: 31580731 Review.
-
Magnetically Driven Micro and Nanorobots.Chem Rev. 2021 Apr 28;121(8):4999-5041. doi: 10.1021/acs.chemrev.0c01234. Epub 2021 Mar 31. Chem Rev. 2021. PMID: 33787235 Free PMC article.
-
Intelligent micro/nanorobots based on biotemplates.Mater Horiz. 2024 Jun 17;11(12):2772-2801. doi: 10.1039/d4mh00114a. Mater Horiz. 2024. PMID: 38597188 Review.
-
Biocompatible smart micro/nanorobots for active gastrointestinal tract drug delivery.Expert Opin Drug Deliv. 2023 Jul-Dec;20(10):1427-1441. doi: 10.1080/17425247.2023.2270915. Epub 2023 Oct 30. Expert Opin Drug Deliv. 2023. PMID: 37840310 Review.
Cited by
-
Micro/Nanosystems for Magnetic Targeted Delivery of Bioagents.Pharmaceutics. 2022 May 26;14(6):1132. doi: 10.3390/pharmaceutics14061132. Pharmaceutics. 2022. PMID: 35745705 Free PMC article. Review.
-
Spermbots and Their Applications in Assisted Reproduction: Current Progress and Future Perspectives.Int J Nanomedicine. 2024 May 31;19:5095-5108. doi: 10.2147/IJN.S465548. eCollection 2024. Int J Nanomedicine. 2024. PMID: 38836008 Free PMC article. Review.
-
Surface-active microrobots can propel through blood faster than inert microrobots.PNAS Nexus. 2024 Oct 15;3(10):pgae463. doi: 10.1093/pnasnexus/pgae463. eCollection 2024 Oct. PNAS Nexus. 2024. PMID: 39474503 Free PMC article.
-
Recent Advances in Electrospinning Techniques for Precise Medicine.Cyborg Bionic Syst. 2024 May 22;5:0101. doi: 10.34133/cbsystems.0101. eCollection 2024. Cyborg Bionic Syst. 2024. PMID: 38778878 Free PMC article. Review.
-
Smart dental materials for antimicrobial applications.Bioact Mater. 2022 Dec 9;24:1-19. doi: 10.1016/j.bioactmat.2022.12.002. eCollection 2023 Jun. Bioact Mater. 2022. PMID: 36582351 Free PMC article.
References
-
- Feynman R. P. There's plenty of room at the bottom. Engineering and Science . 1960;23:22–36.
-
- Sitti M. Voyage of the microrobots. Nature . 2009;458:1121–1122. - PubMed
-
- Erkoc P., Yasa I. C., Ceylan H., Yasa O., Alapan Y., Sitti M. Mobile microrobots for active therapeutic delivery. Advanced Therapeutics . 2019;2, article 1800064 doi: 10.1002/adtp.201800064. - DOI
-
- Ceylan H., Yasa I. C., Kilic U., Hu W., Sitti M. Translational prospects of untethered medical microrobots. Progress in Biomedical Engineering . 2019;1, article 012002
Publication types
LinkOut - more resources
Full Text Sources