Driving axon regeneration by orchestrating neuronal and non-neuronal innate immune responses via the IFNγ-cGAS-STING axis
- PMID: 36370710
- PMCID: PMC9851977
- DOI: 10.1016/j.neuron.2022.10.028
Driving axon regeneration by orchestrating neuronal and non-neuronal innate immune responses via the IFNγ-cGAS-STING axis
Abstract
The coordination mechanism of neural innate immune responses for axon regeneration is not well understood. Here, we showed that neuronal deletion of protein tyrosine phosphatase non-receptor type 2 sustains the IFNγ-STAT1 activity in retinal ganglion cells (RGCs) to promote axon regeneration after injury, independent of mTOR or STAT3. DNA-damage-induced cGAMP synthase (cGAS)-stimulator of interferon genes (STINGs) activation is the functional downstream signaling. Directly activating neuronal STING by cGAMP promotes axon regeneration. In contrast to the central axons, IFNγ is locally translated in the injured peripheral axons and upregulates cGAS expression in Schwann cells and infiltrating blood cells to produce cGAMP, which promotes spontaneous axon regeneration as an immunotransmitter. Our study demonstrates that injured peripheral nervous system (PNS) axons can direct the environmental innate immune response for self-repair and that the neural antiviral mechanism can be harnessed to promote axon regeneration in the central nervous system (CNS).
Keywords: PTPN2; STAT1; axon regeneration; cGAS-STING; dorsal root ganglions; interferon gamma; optic nerve injury; retinal ganglion cells.
Copyright © 2022 The Author(s). Published by Elsevier Inc. All rights reserved.
Conflict of interest statement
Declaration of interests The authors declare no competing interests.
Figures
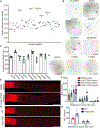
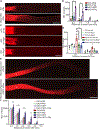
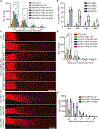
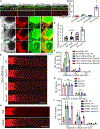
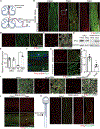
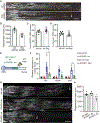
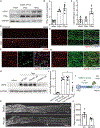
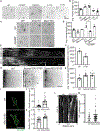
Comment in
-
Antiviral immunity rewired for axon regeneration.Cell Rep Med. 2023 Jan 17;4(1):100907. doi: 10.1016/j.xcrm.2022.100907. Cell Rep Med. 2023. PMID: 36652916 Free PMC article.
Similar articles
-
Antiviral immunity rewired for axon regeneration.Cell Rep Med. 2023 Jan 17;4(1):100907. doi: 10.1016/j.xcrm.2022.100907. Cell Rep Med. 2023. PMID: 36652916 Free PMC article.
-
Retinal ganglion cell survival and axon regeneration after optic nerve injury in naked mole-rats.J Comp Neurol. 2017 Feb 1;525(2):380-388. doi: 10.1002/cne.24070. Epub 2016 Jul 17. J Comp Neurol. 2017. PMID: 27350178 Free PMC article.
-
Receptor protein tyrosine phosphatase sigma inhibits axon regrowth in the adult injured CNS.Mol Cell Neurosci. 2005 Apr;28(4):625-35. doi: 10.1016/j.mcn.2004.10.011. Mol Cell Neurosci. 2005. PMID: 15797710
-
Role of Schwann cells in retinal ganglion cell axon regeneration.Prog Retin Eye Res. 2000 Mar;19(2):171-204. doi: 10.1016/s1350-9462(99)00010-5. Prog Retin Eye Res. 2000. PMID: 10674707 Review.
-
Return of function after CNS axon regeneration: Lessons from injury-responsive intrinsically photosensitive and alpha retinal ganglion cells.Prog Retin Eye Res. 2019 Jul;71:57-67. doi: 10.1016/j.preteyeres.2018.11.006. Epub 2018 Nov 17. Prog Retin Eye Res. 2019. PMID: 30458239 Review.
Cited by
-
Transcriptome sequencing promotes insights on the molecular mechanism of SKP-SC-EVs mitigating denervation-induced muscle atrophy.Mol Biol Rep. 2023 Dec 12;51(1):9. doi: 10.1007/s11033-023-08952-x. Mol Biol Rep. 2023. PMID: 38085347
-
Metformin normalizes mitochondrial function to delay astrocyte senescence in a mouse model of Parkinson's disease through Mfn2-cGAS signaling.J Neuroinflammation. 2024 Apr 2;21(1):81. doi: 10.1186/s12974-024-03072-0. J Neuroinflammation. 2024. PMID: 38566081 Free PMC article.
-
Immune drivers of pain resolution and protection.Nat Immunol. 2024 Dec;25(12):2200-2208. doi: 10.1038/s41590-024-02002-9. Epub 2024 Nov 11. Nat Immunol. 2024. PMID: 39528810 Review.
-
Mechanism and therapeutic potential of targeting cGAS-STING signaling in neurological disorders.Mol Neurodegener. 2023 Nov 8;18(1):79. doi: 10.1186/s13024-023-00672-x. Mol Neurodegener. 2023. PMID: 37941028 Free PMC article. Review.
-
Preparation of Viable Human Neurites for Neurobiological and Neurodegeneration Studies.Cells. 2024 Jan 27;13(3):242. doi: 10.3390/cells13030242. Cells. 2024. PMID: 38334634 Free PMC article.
References
-
- Neumann S, and Woolf CJ (1999). Regeneration of dorsal column fibers into and beyond the lesion site following adult spinal cord injury. Neuron 23, 83–91. - PubMed
Publication types
MeSH terms
Substances
Grants and funding
LinkOut - more resources
Full Text Sources
Molecular Biology Databases
Research Materials
Miscellaneous